《药物化学》课程文献资料(Medicinal Chemistry)Epigenetic protein families - a new frontier for drug discovery
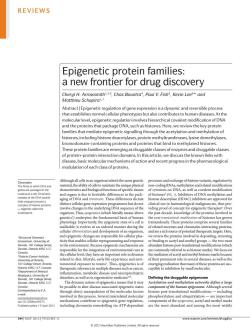
REVIEWS Epigenetic protein families: a new frontier for drug discovery Cheryl H.Arrowsmith,Chas Bountra,Paul V.Fish,Kevin Leesand Matthieu Schapira'. Abstract Epigenetic regulation of gene expression is a dynamic and reversible process that establishes normal cellular phenotypes but also contributes to human diseases.At the molecular level,epigenetic regulation involves hierarchical covalent modification of DNA and the proteins that package DNA,such as histones.Here,we review the key protein families that mediate epigenetic signalling through the acetylation and methylation of histones,including histone deacetylases,protein methyltransferases,lysine demethylases bromodomain-containing proteins and proteins that bind to methylated histones. These p otein families。 protein protein intera domains.n thisarticle, ase,basic mo ar mechanisms of action and modulation of each class of proteins. material,the ability ctions of spec on DNA l.Chr aemato the past decade dge of the】 erpins the hun the post er during the of related s and chro opr arean lular rer ons s ar ne post hey have an in mportant roe in diseas elated t ce a of their prominent ro peuti relevan multiple c Defining the druggable epigenome The ym nature of epigen s that it may Acetylation nd cular factor histone post-translational nvol ed in this proc s.Several interrelated mo phosphorylation and ubiquitylationare importan including chromatin remodelling via ATP-dependent are the most abundant and among the most widely 384 MAY 2012 VOLUME 11 www.nature.com/reviews/drugdisc 2012 Macmillan Publishers Limited.All riehts reserved
Although all cells in an organism inherit the same genetic material, the ability of cells to maintain the unique physical characteristics and biological functions of specific tissues and organs is due to heritable differences in the packaging of DNA and chromatin. These differences dictate distinct cellular gene expression programmes but do not involve changes in the underlying DNA sequence of the organism. Thus, epigenetics (which literally means ‘above genetics’) underpins the fundamental basis of human physiology. Importantly, the epigenetic state of a cell is malleable; it evolves in an ordered manner during the cellular differentiation and development of an organism, and epigenetic changes are responsible for cellular plasticity that enables cellular reprogramming and response to the environment. Because epigenetic mechanisms are responsible for the integration of environmental cues at the cellular level, they have an important role in diseases related to diet, lifestyle, early life experience and environmental exposure to toxins1 . Thus, epigenetics is of therapeutic relevance in multiple diseases such as cancer, inflammation, metabolic disease and neuropsychiatric disorders, as well as in regenerative medicine2–4. The dynamic nature of epigenetics means that it may be possible to alter disease-associated epigenetic states through direct manipulation of the molecular factors involved in this process. Several interrelated molecular mechanisms contribute to epigenetic gene regulation, including chromatin remodelling via ATP-dependent processes and exchange of histone variants, regulation by non-coding RNAs, methylation and related modifications of cytosines on DNA, as well as covalent modification of histones5 (FIG. 1). Inhibitors of DNA methylation and histone deacetylase (HDAC) inhibitors are approved for clinical use in haematological malignancies, thus providing proof of concept for epigenetic therapies6 . Over the past decade, knowledge of the proteins involved in the post-translational modification of histones has grown tremendously. These proteins comprise several families of related enzymes and chromatin-interacting proteins, and are a rich source of potential therapeutic targets. Here, we review the proteins involved in depositing, removing or binding to acetyl and methyl groups — the two most abundant histone post-translational modifications (which are commonly referred to as histone marks). We focus on the mediators of acetyl and methyl histone marks because of their prominent role in several diseases, as well as the emerging realization that many of these proteins are susceptible to inhibition by small molecules. Defining the druggable epigenome Acetylation and methylation networks define a large component of the human epigenome. Although several histone post-translational modifications — including phosphorylation and ubiquitylation — are important components of the epigenome, acetyl and methyl marks are the most abundant and among the most widely 1Structural Genomics Consortium, University of Toronto, 101 College Street, Toronto, Ontario M5G 1L7, Canada. 2Ontario Cancer Institute, University of Toronto, 101 College Street, Toronto, Ontario M5G 1L7, Canada. 3Department of Medical Biophysics, University of Toronto, 101 College Street, Toronto, Ontario M5G 1L7, Canada. Correspondence to C.A. e-mail: carrow@uhnresearch.ca doi:10.1038/nrd3674 Published online 13 April 2012 Epigenetic protein families: a new frontier for drug discovery Cheryl H. Arrowsmith1,2,3, Chas Bountra4, Paul V. Fish5, Kevin Lee6* and Matthieu Schapira1,7 Abstract | Epigenetic regulation of gene expression is a dynamic and reversible process that establishes normal cellular phenotypes but also contributes to human diseases. At the molecular level, epigenetic regulation involves hierarchical covalent modification of DNA and the proteins that package DNA, such as histones. Here, we review the key protein families that mediate epigenetic signalling through the acetylation and methylation of histones, including histone deacetylases, protein methyltransferases, lysine demethylases, bromodomain-containing proteins and proteins that bind to methylated histones. These protein families are emerging as druggable classes of enzymes and druggable classes of protein–protein interaction domains. In this article, we discuss the known links with disease, basic molecular mechanisms of action and recent progress in the pharmacological modulation of each class of proteins. Chromatin The fibres in which DNA and genes are packaged in the nucleus of a cell. Chromatin consists of the DNA double helix wrapped around a complex of histone proteins — together called the nucleosome. REVIEWS 384 | MAY 2012 | VOLUME 11 www.nature.com/reviews/drugdisc © 2012 Macmillan Publishers Limited. All rights reserved
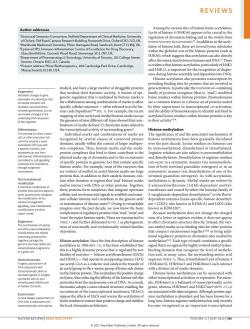
REVIEWS Author addresses 'Structural Genomics Consortium.Nuffield Department of Clinical Medici e to herapeutics.0Cambridge Park Drive.Cambridge. of H4K5 bly and de poNdgbndgss toteinsthatareinoivedih feenec on among cor ature in a diverse set of proteins unit 1).For example,the recent modor the multi- e to th also fpecihcprotcinstoge omic loci that contain For ex mple, of the enzym dino nitrogens)or i terminal guanidino nitr ns).As with acetylation Dost-ra read sone mark methylation is dynamic. SAM ark stream xoglutarate-depe s or the I nce ofd states n as KDMIA)and LSD2 (also onal s are often ade the of the marks.The e the charged ysine or arginine residu loes no as br of proteins. hat compa omes together or bring ins to ee t on n i n 1964 (REF.12),it has b ed tha ignal that is recogniz y highly evolved methyl-lysine of en nd HD r.HAT 1).Thus,trir thylated Lys ofhistone3 each interac rudes from the nucleo ple,H3K4me3 is a hallmark of transcriptionally active atin ad pts a more relaxe genes,wherea K9me3 and H3 27me3 (RE 23.2 se the effects of HATs and reverse the ace tylation of nine methylation is abundant and has been known for es to rest their positive charge and stabiliz NATURE REVIEWSIDRUG DISCOVERY VOLUME 11 MAY 2012 385
Epigenetics Heritable changes in gene expression or phenotype that are stable between cell divisions, and sometimes between generations, but do not involve changes in the underlying DNA sequence of the organism. Differentiation The process by which a stem cell, or other precursor cell, commits towards a more specialized cell type with a specific function, and represents an exit from self-renewal. Differentiation is controlled by cell signalling pathways and maintained through epigenetic mechanisms. Post-translational modification A chemical modification of proteins that acts as a signal to other proteins that recognize the modification. In the context of epigenetic signalling, post-translational modifications are often called ‘marks’. Epigenome The combination of histone and DNA post-translational modifications and related interacting proteins that together package the genome and help define the transcriptional programme in a given cell. Heterochromatin A tightly packed form of DNA associated with transcriptionally silent or repressed genes. It is highly correlated with di- and trimethlyated H3K9 (Lys9 of histone 3) marks. Euchromatin A more loosely packed form of DNA that is associated with transcriptionally active genes. studied, and have a large number of druggable proteins that mediate their dynamic activity. A feature of epigenetic regulation that is mediated by histone marks is the collaboration among combinations of marks to affect specific cellular outcomes — often referred to as the histone code hypothesis7–10 (FIG. 1). For example, the recent mapping of nine acetyl and methyl histone marks across the genomes of nine different cell types showed that combinations of marks defined 15 chromatin states related to the transcriptional activity of surrounding genes11. Individual marks and combinations of marks are recognized by several classes of conserved protein domains, usually within the context of larger multiprotein complexes. Thus, histone marks and the multiprotein complexes that bind to them contribute to the physical make-up of chromatin and to the recruitment of specific proteins to genomic loci that contain specific histone marks. For example, most of the enzymes that are ‘writers’ of methyl or acetyl histone marks are large proteins that, in addition to their catalytic domain, contain other domains or regions that ‘read’ histone marks and/or interact with DNA or other proteins. Together, these proteins form complexes that integrate upstream cellular and environmental signals to establish and maintain cellular identity and contribute to the genesis and/ or maintenance of disease states10. Owing to remarkable progress over the past decade, we now know the basic complement of regulatory proteins that ‘read’, ‘write’ and ‘erase’ the major histone marks. These are summarized in TABLE 1, and further delineated in FIG. 2 as phylogenetic trees of structurally and evolutionarily related families of proteins. Histone acetylation. Since the first description of histone acetylation in 1964 (REF. 12), it has been established that this is a highly dynamic process that is regulated by two families of enzymes — histone acetyltransferases (HATs) and HDACs — that operate in an opposing manner. HATs use acetyl-CoA as a cofactor and catalyse the transfer of an acetyl group to the ε-amino group of lysine side chains on the histone protein. This neutralizes the positive charge on lysine, thus reducing the affinity of the histone tail that protrudes from the nucleosome core of DNA. As a result, chromatin adopts a more relaxed structure, enabling the recruitment of the transcriptional machinery. HDACs oppose the effects of HATs and reverse the acetylation of lysine residues to restore their positive charge and stabilize the local chromatin architecture. Among the various sites of histone lysine acetylation, Lys16 of histone 4 (H4K16) appears to be crucial in the regulation of chromatin folding and in the switch from heterochromatin to euchromatin13. In addition to the acetylation of histone tails, there are several lysine substrates within the globular core of the histone proteins (such as H3K56), which suggests that acetylation can also directly affect the interaction between histones and DNA14. There is evidence that histone acetylation, particularly of H4K5 and H4K12, is important for the recognition of chaperones during histone assembly and deposition into DNA. Histone acetylation also promotes transcription by providing binding sites for proteins that are involved in gene activation. In particular, the bromodomain-containing family of proteins recognize (that is, ‘read’) modified lysine residues within histone proteins. Bromodomains are a common feature in a diverse set of proteins united by their importance in transcriptional co-activation, and the ability of bromodomains to identify and bind to acetylated lysine residues within histone proteins is key to their activity15,16. Histone methylation The significance of and the associated mechanisms of histone methylation have been gradually elucidated over the past decade. Lysine residues on histones can be monomethylated, dimethylated or trimethylated. Arginine residues are also subject to monomethylation and dimethylation. Dimethylation of arginine residues can occur in a symmetric manner (via monomethylation of both terminal guanidino nitrogens) or in an asymmetric manner (via dimethylation of one of the terminal guanidino nitrogens). As with acetylation, methylation is dynamic. Methyl marks are written by S-adenosylmethionine (SAM)-dependent methyltransferases and erased by either the Jumonji family of 2-oxoglutarate-dependent demethylases17 or the flavindependent enzymes lysine-specific histone demethylase 1 (LSD1; also known as KDM1A) and LSD2 (also known as KDM1B)18. Because methylation does not change the charged state of a lysine or arginine residue, it does not appear to effect chromatin structure directly. Instead, the various methyl marks act as binding sites for other proteins that compact nucleosomes together19,20 or bring additional regulatory proteins to chromatin sites marked by methylation21,22. Each type of mark constitutes a specific signal that is recognized by highly evolved methyl-lysinebinding domains that recognize the level of methylation and, in many cases, the surrounding amino acid sequence (TABLE 1). Thus, trimethylated Lys4 of histone 3 (H3K4me3), H3K9me3 and H4K20me2 each interact with a distinct set of reader domains. Histone lysine methylation can be associated with either transcriptional activation or repression. For example, H3K4me3 is a hallmark of transcriptionally active genes, whereas H3K9me3 and H3K27me3 (REFS 23,24) are associated with silenced genes. Although protein arginine methylation is abundant and has been known for a long time, histone arginine methylation has only recently become recognized as an important transcriptional Author addresses 4 Structural Genomics Consortium, Nuffield Department of Clinical Medicine, University of Oxford, Old Road Campus Research Building, Roosevelt Drive, Oxford OX3 7LD, UK. 5 Worldwide Medicinal Chemistry, Pfizer, Ramsgate Road, Sandwich, Kent CT13 9NJ, UK. 6 Epinova DPU, Immuno-Inflammation Centre of Excellence for Drug Discovery, GlaxoSmithKline, Gunnels Wood Road, Stevenage SG1 2NY, UK. 7 Department of Pharmacology & Toxicology, University of Toronto, 101 College Street, Toronto, Ontario M5G 1L7, Canada. *Present address: Pfizer Biotherapeutics, 200 Cambridge Park Drive, Cambridge, Massachusetts 02140, USA. REVIEWS NATURE REVIEWS | DRUG DISCOVERY VOLUME 11 | MAY 2012 | 385 © 2012 Macmillan Publishers Limited. All rights reserved
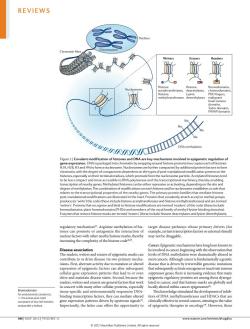
REVIEWS dorpaomin //y igure 1 Covale n of his d in epige eachaationo .H2B.H oteinfactostoioe tivating.dep on the site al prope ry prote diate histone the cod e acety ases and histone m and an pla s)and m ers of th of methyl-lysir ng domains the inte been knownt evels of DNA e dramatically altered ir most cr ougn cancer is amenta ssion of epigenetic factors n alter subsequent that inactivatetumou in canc This kno ent ofinhib. the latter case offers the opportunity to 386 MAY 2012 VOLUME 11 www.nature.com/reviews/drugdisc 2012 Macmillan Publishers Limited.All riehts reserved
Nucleus Writers Erasers Readers Nature Reviews | Drug Discovery Histone acetyltransferases, Histone methyltransferases Histone deacetylases, Lysine demethylases Bromodomains, chromodomains, PHD fingers, malignant brain tumour domains, Tudor domains, PWWP domains DNA methylation Me Me Me Me Chromatin fibre Chromosome Nucleosome Bromodomain An evolutionarily conserved, ~110-amino-acid motif composed of four left-handed, antiparallel α-helices. regulatory mechanism25. Arginine methylation of histones can promote or antagonize the interaction of nuclear factors with other nearby histone marks, thereby increasing the complexity of the histone code26,27. Disease association The readers, writers and erasers of epigenetic marks can contribute to or drive disease via two primary mechanisms. First, aberrant activity due to mutation or altered expression of epigenetic factors can alter subsequent cellular gene expression patterns that lead to or even drive and maintain disease states. Second, because the readers, writers and erasers are general factors that work in concert with many other cellular proteins, especially tissue-specific and environmentally responsive DNAbinding transcription factors, they can mediate altered gene expression patterns driven by upstream signals10. Importantly, the latter case offers the opportunity to target disease pathways whose primary drivers (for example, certain transcription factors or external stimuli) may not be druggable. Cancer. Epigenetic mechanisms have long been known to be involved in cancer, beginning with the observation that levels of DNA methylation were dramatically altered in most cancers. Although cancer is fundamentally a genetic disease that is driven by irreversible genomic mutations that subsequently activate oncogenes or inactivate tumour suppressor genes, there is increasing evidence that many epigenetic regulatory proteins are among those dysregulated in cancer, and that histone marks are globally and locally altered within cancer epigenomes28. This knowledge stimulated the development of inhibitors of DNA methyltransferases and HDACs that are clinically effective in several cancers, attesting to the value of epigenetic therapies in oncology28. However, these Figure 1 | Covalent modification of histones and DNA are key mechanisms involved in epigenetic regulation of gene expression. DNA is packaged into chromatin by wrapping around histone proteins (two copies each of histones H2A, H2B, H3 and H4) to form a nucleosome. Nucleosomes are further compacted by additional protein factors to form chromatin, with the degree of compactness dependent on the types of post-translational modification present on the histones, especially on their terminal residues, which protrude from the nucleosome particle. Acetylated histones tend to be less compact and more accessible to RNA polymerase and the transcriptional machinery, thereby enabling transcription of nearby genes. Methylated histones can be either repressive or activating, depending on the site and degree of methylation. The combination of modifications on each histone and/or nucleosome establishes a code that relates to the transcriptional properties of the nearby genes. The primary protein families that mediate histone post-translational modifications are illustrated in the inset. Proteins that covalently attach acetyl or methyl groups produce (or ‘write’) the code (these include histone acetyltransferases and histone methyltransferases) and are termed ‘writers’. Proteins that recognize and bind to histone modifications are termed ‘readers’ of the code (these include bromodomains, plant homeodomains (PHDs) and members of the royal family of methyl-lysine-binding domains). Enzymes that remove histone marks are termed ‘erasers’ (these include histone deacetylases and lysine demethylases). REVIEWS 386 | MAY 2012 | VOLUME 11 www.nature.com/reviews/drugdisc © 2012 Macmillan Publishers Limited. All rights reserved
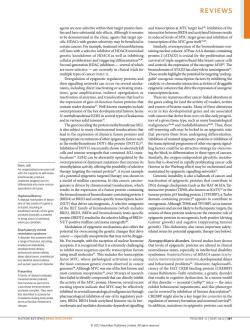
REVIEWS gh it remains MYCtarget of the con oftiple-negative/b ATAD2 has a key role in tumorig their signalling netw can oc tion activities of drugg ngoractivat ind nd tra hecapreserdo r20 on of the key developmental his r in key dev I are associate ofgiven tissu 山as man ignancies nd m Thus,thes lead to the exp ngdcrcntiatio Inhibition of DOTILwasre om and t ng- tion in th ion of that inc ncer Stem cell bility of sel may be orch strated and alzedpmecusorcel nethylation activityoffering the pos of a p netic targeted the rapy was shown in eny)an arcinoma.In this tion protei ture I type sults in the exp TIP60:also kno a所cin ing pro 3BP1; oncogene and TP53BP1 act as tum 3RD2.BRD3.BRD4 and b targe in This ve suppressing tun oming the ger etic changes that driv dis rs,it is recognized that i s extremely chall hat lev eins are altered in clin the tran rodisease states,epec nd s r MYC whose pathological activatio is among synd e,developmental de and autism spectrum disorde n and the HAT CREB binding protein (CREBBP auses Rubinstein- Taybi syndrome he activity of the mYc Mein Ho of this dis onatal crehhe mi the mice reports ndicat hat M may be effectiv xhibit behavi pairme fhe and is ph har ical inhibition of one of its regulato art CREBBP might also be a key target for presen n the 4 binds cetylat s mer n epigenetic pr NATURE REVIEWSIDRUG DISCOVERY VOLUME 11 MAY 2012 387
Stem cell An unspecialized precursor cell with the capacity to self-renew (continuously produce unaltered progeny) and to differentiate into more mature specialized cell types. Haploinsufficiency A disease mechanism in which one of two copies of a gene is mutated, resulting in insufficient activity of the gene products (typically a protein) to bring about a functional, wild-type condition. Brachydactyly mental retardation syndrome A disorder that presents with a range of features, including intellectual disabilities, developmental delays, behavioural abnormalities, sleep disturbance, craniofacial and skeletal abnormalities, and autism spectrum disorder. Presenilins A family of related multipass transmembrane proteins that function as part of the γ-secretase intramembrane protease complex. They were first identified in screens for mutations causing early-onset forms of familial Alzheimer’s disease. agents are non-selective within their target protein families and have substantial side effects. Although it remains to be demonstrated in the clinic, agents that target specific HDACs with greater selectivity may be beneficial in certain cancers. For example, treatment of neuroblastoma cell lines with a selective inhibitor of HDAC8 mimicked genetic knockdown of HDAC8 as well as inhibiting cellular proliferation and triggering differentiation29,30. Second-generation HDAC inhibitors — several of which are more selective — are currently in clinical trials for multiple types of cancer (TABLE 2). Deregulation of epigenetic regulatory proteins and their signalling networks can occur via several mechanisms, including direct inactivating or activating mutations, gene amplification, indirect upregulation or inactivation of enzymes, and translocations that lead to the expression of gain-of-function fusion proteins that contain reader domains31. Well-known examples include overexpression of the key developmental histone lysine N-methyltransferase EZH2 in several types of leukaemia and in various solid tumours32. The gene encoding the protein methyltransferase MLL is also subject to many chromosomal translocations that lead to the expression of chimeric fusion proteins and inappropriate recruitment of other epigenetic factors such as the methyltransferase DOT1-like protein (DOT1L)33. Inhibition of DOT1L was recently shown to selectively kill cells and tumour xenografts that contained MLL translocations34. EZH2 can be aberrantly upregulated by the overexpression of dominant mutations that increase its trimethylation activity, offering the possibility of selective therapy targeting the mutant protein35. A recent example of a potential epigenetic targeted therapy was shown in a model of midline carcinoma. In this cancer, carcinogenesis is driven by chromosomal translocation, which results in the expression of a fusion protein containing the bromodomain of bromodomain-containing protein 4 (BRD4) or BRD3 and a testis-specific transcription factor (NUT) that drives carcinogenesis. A selective antagonist of the BET family of bromodomains (which includes BRD2, BRD3, BRD4 and bromodomain testis-specific protein (BRDT)) resulted in the selective killing of BRD4– NUT-positive midline carcinoma xenografts36. Modulation of epigenetic mechanisms also offers the potential for overcoming the genetic changes that drive cancer — especially oncoproteins that may not be druggable. For example, with the exception of nuclear hormone receptors, it is recognized that it is extremely challenging to inhibit most sequence-specific transcription factors using small molecules37. This includes the transcription factor MYC, whose pathological activation is among the most common genetic events observed in cancer genomes38. Although MYC was one of the first known and most common oncoproteins39, over 30 years of research have failed to identify compounds that can directly inhibit the activity of the MYC protein. However, several recent exciting reports indicate that MYC may be effectively inhibited in several haematological malignancies through pharmacological inhibition of one of its regulatory partners, BRD4. BRD4 binds acetylated histones via its bromodomain and mediates chromatin-dependent signalling and transcription at MYC target loci40. Inhibition of the interaction between BRD4 and acetylated histones results in reduced levels of MYC target genes and inhibition of transcription of the MYC gene itself 41,42. Similarly, overexpression of the bromodomain-containing nuclear cofactor ATPase AAA domain-containing protein 2 (ATAD2) is crucial for the proliferation and survival of triple-negative/basal-like breast cancer cells and controls the expression of the oncogene MYB43. The bromodomain of ATAD2 has a key role in tumorigenesis44. These results highlight the potential for targeting ‘undruggable’ oncogenic transcription factors by inhibiting the catalytic or chromatin-interaction activities of druggable epigenetic cofactors that drive the expression of oncogenic transcription factors. There are numerous other cancer-linked alterations in the genes coding for (and the activity of) readers, writers and erasers of histone marks. Many of these alterations occur in key developmental genes and are associated with cancers that derive from stem cell-like early progenitors of a given tissue type, such as many haematological malignancies45–48 and medulloblastoma49,50. Thus, these self-renewing cells may be locked in an epigenetic state that prevents them from undergoing differentiation. Inhibition of mutated epigenetic proteins or inhibition of the transcriptional programme of other oncogenic signalling factors could be an attractive strategy for overcoming the block to differentiation in these types of cancers. Similarly, the oxygen-independent glycolytic metabolism that is observed in rapidly proliferating cancer cells (known as the Warburg effect) may be orchestrated and maintained by epigenetic signalling networks51. Genomic instability is also a hallmark of cancer, and inactivation of epigenetic proteins that contribute to DNA damage checkpoints (such as the HAT 60 kDa Tatinteractive protein (TIP60; also known as KAT5)52 or the tumour protein p53 binding protein 1 (TP53BP1; a Tudor domain-containing protein)53 appears to contribute to oncogenesis. Although TIP60 and TP53BP1 act as tumour suppressors and are not likely to be therapeutic targets, the actions of these proteins underscore the extensive role of epigenetic proteins in oncogenesis, both positive (driving tumour growth) and negative (suppressing tumour growth). This dichotomy also raises important safetyrelated issues for potential epigenetic therapy (see below). Neuropsychiatric disorders. Several studies have shown that levels of epigenetic proteins are altered in clinical neurodisease states, especially in intellectual disability syndromes. Haploinsufficiency of HDAC4 causes brachydactyly mental retardation syndrome, developmental delays and behavioural problems54. Moreover, haploinsufficiency of the HAT CREB binding protein (CREBBP) causes Rubinstein–Taybi syndrome, a genetic disorder that results in cognitive dysfunction. In a mouse model of this disorder — neonatal Crebbp+/– mice — the mice exhibit behavioural impairments, and this phenotype can be reversed by inhibition of histone deacetylation55. CREBBP might also be a key target for presenilins in the regulation of memory formation and neuronal survival56. In addition, mutations in epigenetic proteins can result REVIEWS NATURE REVIEWS | DRUG DISCOVERY VOLUME 11 | MAY 2012 | 387 © 2012 Macmillan Publishers Limited. All rights reserved
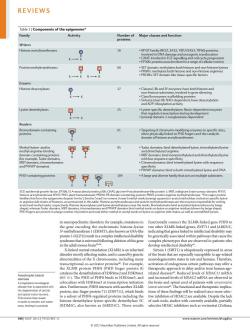
REVIEWS Table1Components of the epigenome Family Activity Major classes and function Writers Histone acetyltransferases 18 Protein methvitransferases 60 ctor Erasers Histone deacetylases 9 Lysine demethylases 25 K-k Readers proteins main-containing F in of histone ac Methy-nn 可可 ndeordemaiebradimcgthnytatedbsine,timethtatedbysine aining.g8 mains:bind toboth trimethylated lysine and DNA PHD-containing proteins 104 A large and diverse family that acts on multiple substrates m th bind to or ac blue circles)o in a large of p 0choaihintoneyin the XLMR-linked (EHMTI: ectua y notypes that patientswho ed in disorder mostly af ding many states in rats and umans.I neretore D finger rotein 8) apeutic appro ach to delay and/or otophiclateral RE u.The PHD of PHE8 binds to H3K4me3.and and in ption the brain and spina ord of pat of cen rotein zine fir tions of these find o a subset of F proteins incuding th 2 are available. aste (KDM5C.also known as IARIDIC)These results selective HDAC inhibitors such as vorinostat (oinz 388 MAY 2012 VOLUME 11 www.nature.com/reviews/drugdisc
Amyotrophic lateral sclerosis A progressive neurological disease that is associated with the degeneration of central and spinal motor neurons. This neuron loss causes muscles to weaken and waste away, leading to paralysis. in neuropsychiatric disorders: for example, mutations in the gene encoding the euchromatic histone-lysine N-methyltransferase 1 (EHMT1; also known as G9A-like protein 1 (GLP1)) result in a complex intellectual disability syndrome that is mirrored following deletion of this gene in the adult mouse brain57–59. X-linked mental retardation (XLMR) is an inherited disorder mostly affecting males, and is caused by genetic abnormalities of the X chromosome, including many transcriptional co-activator proteins60. For example, the XLMR protein PHF8 (PHD finger protein 8) catalyses the demethylation of H3K9me2 and H3K9me1 (REF. 61). The PHD of PHF8 binds to H3K4me3, and colocalizes with H3K4me3 at transcription initiation sites. Furthermore, PHF8 interacts with another XLMR protein, zinc finger protein 711 (ZNF711), which binds to a subset of PHF8-regulated proteins including the histone demethylase lysine-specific demethylase 5C (KDM5C; also known as JARID1C). These results functionally connect the XLMR-linked gene PHF8 to two other XLMR-linked genes, ZNF711 and JARID1C, indicating that genes linked to intellectual disability may be genetically associated within pathways that cause the complex phenotypes that are observed in patients who develop intellectual disability61. Sirtuin 1 (SIRT1) is ubiquitously expressed in areas of the brain that are especially susceptible to age-related neurodegenerative states in rats and humans. Therefore, activation of endogenous sirtuin pathways may offer a therapeutic approach to delay and/or treat human agerelated diseases62. Reduced levels of HDAC11 mRNA and increased levels of HDAC2 mRNA are observed in the brain and spinal cord of patients with amyotrophic lateral sclerosis63. The functional and therapeutic implications of these findings will be realized once more selective inhibitors of HDAC2 are available. Despite the lack of such tools, studies with currently available, partially selective HDAC inhibitors such as vorinostat (Zolinza; Table 1 | Components of the epigenome* Family Activity Number of proteins Major classes and function Writers Histone acetyltransferases K K 18 • MYST family (MOZ, SAS2, YBF2/SAS3, TIP60) proteins: involved in DNA damage and oncogenic translocation • GNAT: involved in EGF signalling and cell cycle progression • EP300: promiscuous (involved in a range of cellular events) Protein methyltransferases K K R R 60 • SET domain: methylates both histone and non-histone lysines • PRMTs: methylate both histone and non-histone arginines • PRDMs: SET domain-like tissue-specific factors Erasers Histone deacetylases K K 17 • Classes I, IIb and IV enzymes: have both histone and non-histone substrates, involved in gene silencing • Class IIa enzymes: scaffolding proteins • Sirtuins (class III): NAD-dependent, have deacetylation and ADP-ribosylation activity Lysine demethylases K K 25 • Lysine-specific demethylases: flavin-dependent enzymes that regulate transcription during development • Jumonji domain: 2-oxoglutarate-dependent Readers Bromodomain-containing proteins K 61 • Targeting of chromatin-modifying enzymes to specific sites, often physically linked to PHD fingers and the catalytic domain of histone acetyltransferases Methyl-lysine- and/or methyl-arginine-binding domain-containing proteins (for example, Tudor domains, MBT domains, chromodomains and PWWP domains) K R 95 • Tudor domains: bind dimethylated lysine, trimethylated lysine and dimethylated arginine • MBT domains: bind monomethylated and dimethylated lysine with low sequence specificity • Chromodomains: bind trimethylated lysine with sequence specificity • PWWP domains: bind to both trimethylated lysine and DNA PHD-containing proteins K R K K 104 • A large and diverse family that acts on multiple substrates EGF, epidermal growth factor; EP300, E1A-associated protein p300; GNAT, glycine-N-acyltransferase-like protein 1; MBT, malignant brain tumour domain; MYST, histone acetyltransferase MYST; PHD, plant homeodomain; PRDM, PR domain-containing protein; PRMT, protein arginine methyltransferase. *The major protein families that form the epigenome deposit (‘write’), bind to (‘read’) or remove (‘erase’) methyl marks (orange squares) or acetyl marks (blue circles) on specific lysine or arginine side chains of histones, as summarized in this table. Histone acetyltransferases and protein methyltransferases are the enzymes responsible for writing acetyl and methyl marks, respectively. Histone deacetylases and lysine demethylases erase the marks. Bromodomains bind acetylated lysines (shown by beige shape), whereas Tudor domains, MBT domains, chromodomains and PWWP domains bind methyl marks on lysine or arginine residues (shown by beige shape). PHD fingers are present in a large number of proteins and read either methyl or acetyl marks on lysine or arginine side chains, as well as unmodified lysines. REVIEWS 388 | MAY 2012 | VOLUME 11 www.nature.com/reviews/drugdisc © 2012 Macmillan Publishers Limited. All rights reserved

REVIEWS in-containing p ein 3(MID also know The gene encoding as KDM6B) been implicated in the reo 0 nt of t ha ms in A2 that are angiogenesi ummar there is a growing body of mole ula m in sclinical evidenceto suggest that these mechanismsn targe The ad exhibit Metabolic disarders The sirtuins which deacetvlate both substrates are majo which then been associated with a lower body mass index in tw nato ctivity of SIRTI has been as "and in diabetes in huma dies have revealed that several specific HDACs are have favourable phv une respons heindirectactne IRT HDAC6 and HDAC9 enhan f the tran rate studyon-em erebro entriculr infusion o box P3 to promo the activit diet-i obe betic mice norm antigen-pres RNA the immun D01 e by SIRT stoe methylatio coatributestomypergyCaerm -tra slational modificati of the e NF-xBp65 sub (SETD7) variegation and by and suppr 63 f th oth through acetylation of h s and th ugh tran the ation of the i mune r ponse Regenerative medicine:role in er nic stem cell differ alyt al in th lysine temic global iclhrynthedirecteddfie iation ofembryonic histone lysine methylation in the ofimmun of induced pluripotent stem cells by rep ammin ch the the protein methyltransteras mportant ted wit silen ment of diabetes and neu as P ns d ted w ith pu NATURE REVIEWSIDRUG DISCOVERY VOLUME 11 MAY 2012 389
Jumonji domain A conserved domain originally identified in the Jumonji family of transcription factors, now known to be histone demethylases. The Jumonji C domain comprises the catalytic domain of the 2-oxoglutarate-dependent lysine demethylases. Hyperglycaemic memory A phenomenon in which the deleterious end-organ effects resulting from exposure to high glucose levels persist for several years after usual glycaemic control is restored. Merck)64 and MS-275 (REF. 65) are revealing great insights into the role of these HDACs in central nervous system pathologies. Schizophrenia is another disorder in which there are altered levels of epigenetic proteins. The gene encoding SMARCA2 (SWI/SNF-related matrix-associated actindependent regulator of chromatin subfamily A member 2) expresses BRM, which is a protein component of the SWI/ SNF chromatin-remodelling complex; this complex has been associated with schizophrenia in genome-wide association studies. Polymorphisms in SMARCA2 that are linked to the disease produce changes in the expression of the gene and/or in the encoded amino acid sequence66. In addition, a polymorphism in BRD1 has been shown to be associated with schizophrenia and bipolar affective disorder67. Inflammation. The adaptive immune response exhibits hallmarks of a system that is subject to epigenetic regulation. The adaptive immune system is composed of multipotent precursor cells that undergo differentiation and clonal expansion upon exposure to an appropriate stimulus (for example, an antigen) to become activated lymphocytes, which then retain a memory against future exposure. It is therefore unsurprising that non-selective HDAC inhibitors have demonstrated preclinical efficacy in several rodent models of inflammatory conditions, both in rodent disease models68 and in clinical samples taken from patients with autoimmune disease69. These studies have revealed that several specific HDACs are implicated in various aspects of the immune response, including the innate and adaptive system70. For example, HDAC6 and HDAC9 enhance the activity of the transcription factor forkhead box P3 to promote the activity of anti-inflammatory regulatory T lymphocytes71, whereas HDAC6 has recently been implicated in the differentiation and maturation of antigen-presenting dendritic cells72. In addition, several sirtuins have been shown to regulate the immune response by modulating the activity of key transcription factors. For example, SIRT1 and SIRT6 modulate nuclear factor-κB (NF-κB) activity via post-translational modification of the NF-κB p65 subunit and by altering the accessibility of the promoters to p65, respectively73. Accordingly, SIRT1 activators have anti-inflammatory effects in in vitro and in vivo models of inflammation74. Several HATs also regulate the inflammatory response, both through acetylation of histones and through transcription factors such as NF-κB75. These findings clearly indicate an important role for acetylation in the regulation of the immune response; this is further supported by recent findings indicating that the BET family of bromodomain-containing proteins is pivotal in the systemic global inflammatory response to endotoxin76. In addition, there is growing evidence of a role for histone lysine methylation in the regulation of immune processes. In particular, the protein methyltransferase G9A (also known as EHMT2) is important in mediating the silencing of specific genes during endotoxin shock via H3K9 dimethylation77. The flavin-dependent amine oxidase LSD2 mediates NF-κB demethylation and, in doing so, it has been implicated in a regulatory circuit that controls the expression of pro-inflammatory genes in dendritic cells78. Moreover, the histone demethylase Jumonji domain-containing protein 3 (JMJD3; also known as KDM6B) has been implicated in the response of macrophages to lipopolysaccharides and in the activation and maintenance of so-called ‘alternatively activated’ macrophages, which are thought to be involved in the host response to parasites, tissue remodelling and angiogenesis79,80. In summary, there is a growing body of molecular and pharmacological evidence that epigenetic machinery is involved in the regulation of the immune system via mechanisms that involve modulation of transcription factors and modification of histones. In addition, there is clinical evidence to suggest that these mechanisms may be deregulated in autoimmune diseases81,82; targeting epigenetic regulators may therefore represent powerful new approaches for the amelioration of these conditions. Metabolic disorders. The sirtuins, which deacetylate both histone and non-histone substrates, are major regulators of metabolism83. Two common variants in SIRT1 have been associated with a lower body mass index in two independent Dutch populations. Carriers of these variants have a reported 13–18% decreased risk of obesity84. Reduced levels or reduced activity of SIRT1 has been associated with complications of type 2 diabetes in humans85 and mice86. Thus, activation of one or more sirtuins can have favourable physiological effects. Resveratrol, through the indirect activation of SIRT1, stimulates insulin release in insulinoma INS-1E cells and human islets87. In a separate study, long-term intracerebroventricular infusion of resveratrol to diet-induced obese diabetic mice normalized hyperglycaemia and improved hyperinsulinaemia88. These effects were also reported to be mediated via SIRT1, as demonstrated by knockdown of the protein using short hairpin RNA89. Histone methylation contributes to hyperglycaemic memory in models of transient hyperglycaemia90. The methyltransferases SET domain-containing protein 7 (SETD7) and suppressor of variegation 3–9 homolog 1 (SUV39H1), as well as the demethylase LSD1, contribute to sustained upregulation of the gene encoding the p65 subunit of NF-κB in response to glucose. Knockdown of SETD7 reverses effects that are associated with diabetic vascular injury, suggesting that this protein lysine methyltransferase (PKMT) is a potential target for the treatment of diabetes91. Regenerative medicine: role in embryonic stem cell differentiation and reprogramming. Modulation of epigenetic proteins has shown utility in regenerative medicine, particularly in the directed differentiation of embryonic stem cells towards a committed lineage, and in the formation of induced pluripotent stem cells by reprogramming somatic cells92. Important opportunities associated with HDAC proteins in regenerative medicine are in the treatment of diabetes and neurodegenerative disorders such as Parkinson’s disease and Alzheimer’s disease. Stem cells treated with putative HDAC inhibitors demonstrated REVIEWS NATURE REVIEWS | DRUG DISCOVERY VOLUME 11 | MAY 2012 | 389 © 2012 Macmillan Publishers Limited. All rights reserved
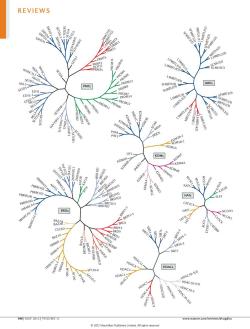
REVIEWS 012) RN L3MB SETD2 1 PMTs EZH2-EZH M1 46 L3MB NA UTY KDMs DM6 DM4A D3(1) PBRM1 MAR BRDs HAT1- OA 1 A CECR -SIRT6-2 SIRT SP110-6 HDACs HDAC10-1(2) a DAC7_ DAC10-10 HDAC10-5 390 MAY 2012 VOLUME 11 www.nature.com/reviews/drugdisc 2012 Macmillan Publishers Limited.All rights reserve
KDMs KDM4C KDM4A KDM4B KDM4DL KDM4D KDM5D KDM5C KDM5A KDM5B KDM6B JARID2 KDM6A UTY PHF2 PHF8 JHDM1D KDM2B KDM2A JMJD1C KDM3A KDM3B NO66 MINA JMJD5 KDM1B-2 KDM1B-1 KDM1A PRMT7(2) PRMT7(1) CARM1 PRMT8 PRMT1 PRMT3 PRMT6 PRMT2 DOT1L PRMT5 PRDM15 PRDM10 PRDM4 PRDM8 PRDM13 PRDM9 PRDM7 PRDM14 PRDM12 PRDM11 PRDM6 PRDM5 PRDM1 MDS1 PRDM16 PRDM2 SETDB2 SETDB1 EHMT1 EHMT2 SUV39H2 SUV39H1 SETMAR SETD1B SETD1A MLL4 MLL MLL3 EZH2-5 MLL2 EZH2-1 SETD5 EZH1 MLL5 SETD2 ASH1L WHSC1L1 WHSC1 SETD8 NSD1 PMTs SETD3 SUV420H2 SUV420H1 SETD7 SETD4 SETD6-1 SETD6-2 SMYD1 SMYD3 SMYD4 SMYD2 SMYD5 Nature Reviews | Drug Discovery SMARCA2-2 BAZ2A BAZ2B CECR2 BAZ1A TRIM66 TRIM24 TRIM33-1 TRIM33-2 TRIM28 SP100 SP140 SP140L SP110-1 SP110-6 SMARCA2-1 SMARCA4 PBRM1(6) PBRM1(4) PBRM1(5) PBRM1(3) PBRM1(1) ASH1L PBRM1(2) TAF1L(2) TAF1L(1) TAF1(1) ZMYND8 BPTF BRD3(2) BRD2(2) BRD4(2) BRDT(2) BRWD1(1) BRWD3(1) BRDT(1) BRD3(1) BRD2(1) BRD4(1) BRPF1-2 BRPF1-1 BRD1 BRPF3 BRD9 ATAD2B BRD7 CREBBP EP300 MLL BRWD3(2) PHIP(2) BRD8(1) BRWD1(2) BRD8(2) BAZ1B ATAD2 PHIP(1) KAT2B KAT2A ZMYND11 TAF1(2) BRDs HDAC10-1(2) HDAC10-1(1) HDAC10-5 HDAC10-2(1) HDAC6(1) HDAC6(2) HDAC9 HDAC5 HDAC4 HDAC7 HDAC2 HDAC1 HDAC3 HDAC8 HDAC11 SIRT7 SIRT6-2 SIRT6-1 SIRT4 SIRT6-4 SIRT5 SIRT3 SIRT1 SIRT2 HDACs KAT5 MYST1 MYST2 MYST3 MYST4 KAT2A KAT2B ELP3 NCOA3 CLOCK NCOA1 CREBBP EP300 ATAT1 TAF1 TAF1L HAT1 GTF3C4 HATs SCMH1(1) SCML2(1) L3MBTL2(2) MBTD1(2) SFMBT2(2) SFMBT1(2) L3MBTL2(1) MBTD1 (1) SFMBT1(1) SFMBT2(1) L3MBTL3(1) L3MBTL(1) L3MBTL4(1) L3MBTL4(3) SFMBT1(4) SFMBT2(4) L3MBTL(3) L3MBTL3(3) L3MBTL2(4) MBTD1(4) L3MBTL(2) L3MBTL4(2) L3MBTL3(2) SCMH1(2) SCML2(2) L3MBTL2(3) MBTD1(3) SFMBT1(3) SFMBT2(3) MBTs REVIEWS 390 | MAY 2012 | VOLUME 11 www.nature.com/reviews/drugdisc © 2012 Macmillan Publishers Limited. All rights reserved
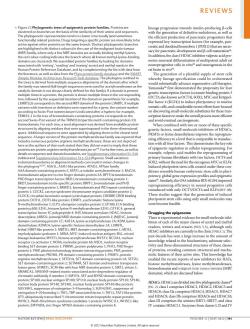
REVIEWS Figure 2|Phyloge etic tree s of epi roteinfhmilies.Protein he phy a tends to cluster st cturall (and sc the 6、 n factors (Pan homeobox 1 (PD )that arer nily.whe e only a f N MBT lly bind sI HDAC inhibitor s the ns are We as ed pr mili in motes neuron n Protein ref nce Datab a the ist with chitec Res med (full-e ially advance re erative cell the 6 ca first ys times ina tim )SOX2,MYC an ds to the nd MBT d the pr ss-Pr icated afte isco ring small-m cule st stitutes for the tran n the theTRIM33( he ov ss mor artit ot ith e of the es b imp sed combined the t .A larg the gram ansfe tive ming effic y to a level tha is comparable to transd did n in cellular re Fo cna the 89 mer methodsca h vithout the need for t nesMYCor KLF4 TAD nduced pluri potent stem s crea 2 bal g files and epigeneti 01294 npro nd WD with onl REF 98 CLOCK ioaoinduce p30 m feasible e:AF om ety ein ce for small-molecule inhi the ma anc ethy thylas rs are cu n the clinic MINA duced nu MYST1:NCOA e amount o rotein 6 d three-dimensional structur s of these cla ro Z:PH P.ple logy ain int ing onta ETMA enabled the recent reports of new inhibitors for HATs h four MB s protein 1: s and mali domains),which are discussed below. YD and MY 140 SP140 40 HDACs.HDACsare dividedin o five ph lear body ike prote 2):class I comprises HD HD TAFI-ike protein DAC 1og 1:d AF1.TBP-as factor 1 and HDAC9;class IIb s HDAC and HDACIO - ate WHSC1L1.WHSC1-like T7ianddas NATURE REVIEWSIDRUG DISCOVERY VOLUME 11 MAY 2012 391
▶ Figure 2 | Phylogenetic trees of epigenetic protein families. Proteins are clustered on branches on the basis of the similarity of their amino acid sequences. The phylogenetic representation tends to cluster structurally (and sometimes functionally) related proteins. Drugs targeting a specific protein are more likely to be active against other proteins on the same branch. Distinct phylogenetic branches are highlighted with distinct colours (in the case of the malignant brain tumour (MBT) family, where only a few MBT domains are actually binding methyl-lysines, the red colour coding indicates the branch where all known methyl-lysine-binding domains are clustered). We assembled protein families by looking for domains associated with ‘writing’, ‘reading’ and ‘erasing’ acetyl and methyl marks in the Human Protein Reference Database, and by complementing the list with data from the literature, as well as data from the Pfam protein family database and the SMART (Simple Modular Architecture Research Tool) database. The phylogeny outlined in the trees is derived from multiple sequence alignments of the domain after which the family was named (full-length sequences were used for acetyltransferases as the catalytic domain is not always clearly defined for this family). If a domain is present multiple times in a protein, the protein is shown multiple times in the corresponding tree, followed by the sequential iteration of the domain in parenthesis: for example, L3MBTL(2) corresponds to the second MBT domain of the protein L3MBTL. If multiple variants with insertions or deletions were reported for a gene, the variant number according to Swiss-Prot nomenclature is indicated after a hyphen: for example, TRIM33-2 in the tree of bromodomain-containing proteins corresponds to the second Swiss-Prot variant of the TRIM33 (tripartite motif-containing protein 33) bromodomain. For each tree, a seed alignment was derived from available protein structures by aligning residues that were superimposed in the three-dimensional space. Additional sequences were appended by aligning them to the closest seed sequence. A larger version of the protein methyltransferase family was reported that includes numerous putative arginine methyltransferases; these are not depicted here as the authors of that work stated that they did not want to imply that these proteins are protein arginine methyltransferases per se173. For further trees, as well as details on sequence and domain boundaries, see Supplementary information S1–S10 (tables) and Supplementary information S11–S14 (figures). Small variations in domain boundaries or alignment methods can result in minor changes in the phylogeny15,173. ASH1L, ASH1-like protein; ATAD2, ATPase family AAA domain-containing protein 2; ATAT1, α-tubulin acetyltransferase 1; BAZ2A, bromodomain adjacent to zinc finger domain protein 2A; BPTF, bromodomain PHD finger transcription factor; BRD1, bromodomain containing protein 1; BRDT, bromodomain testis-specific protein; BRPF1, bromodomain and PHD finger-containing protein 1; BRWD1, bromodomain and WD repeat-containing protein 1; CECR2, cat eye syndrome chromosome region candidate protein 2; CLOCK, circadian locomoter output cycles kaput protein; CREBBP, CREB binding protein; DOT1L, DOT1-like protein; EHMT1, euchromatic histone lysine N-methyltransferase 1; ELP3, elongator complex protein 3; EP300, E1A binding protein p300; EZH1, histone lysine N-methyltransferase EZH1; GTF3C4, general transcription factor 3C polypeptide 4; HAT, histone acetylase; HDAC, histone deacetylase; JARID2, Jumonji/ARID domain-containing protein 2; JMJD1C, Jumonji domain-containing protein 1C; KAT2A, lysine acetyltransferase 2A; KDM, lysine demethylase; KDM1A, lysine-specific histone demethylase 1A; L3MBTL, lethal 3 MBT-like protein 1; MBTD1, MBT domain-containing protein 1; MDS1, myelodysplasia syndrome 1; MINA, MYC-induced nuclear antigen; MLL, mixed lineage leukaemia; MYST1, histone acetyltransferase MYST1; NCOA1, nuclear receptor co-activator 1; NO66, nucleolar protein 66; NSD1, nuclear receptor binding SET domain protein 1; PBRM1, protein polybromo 1; PHF2, PHD finger protein 2; PHIP, pleckstrin homology domain interacting protein; PMT, protein methyltransferase; PRDM1, PR domain-containing protein 1; PRMT1, protein arginine methyltransferase 1; SETD1A, SET domain containing protein 1A; SETD2, SET domain-containing protein 2; SETMAR, SET domain and mariner transposase fusion gene; SFMBT1, SCM-like with four MBT domains protein 1; SIRT1, sirtuin 1; SMARCA2, SWI/SNF-related matrix-associated actin-dependent regulator of chromatin subfamily A member 2; SMYD1, SET and MYND domain-containing protein; SP100, nuclear antigen SP100; SP110, nuclear body protein SP110; SP140, nuclear body protein SP140; SP140L, nuclear body protein SP140‑like protein; SUV39H1, suppressor of variegation 3–9 homolog 1; SUV420H1, suppressor of variegation 4–20 homolog 1; TAF1, TBP-associated factor 1; TAF1L, TAF1‑like protein; UTY, ubiquitously transcribed Y chromosome tetratricopeptide repeat protein; WHSC1, Wolf–Hirschhorn syndrome candidate 1 protein; WHSC1L1, WHSC1-like protein; ZMYND8, zinc finger MYND domain-containing protein 8. lineage progression towards insulin-producing β-cells with the generation of definitive endoderms, as well as the efficient production of pancreatic progenitors that expressed key transcription factors (for example, pancreatic and duodenal homeobox 1 (PDX1)) that are necessary for pancreatic development and β-cell maturation93. In addition, the class I HDAC inhibitor valproic acid promotes neuronal differentiation of multipotent adult rat neuroprogenitor cells in vitro94 and neurogenesis in the rat brain in vivo95. The generation of a plentiful supply of stem cells whereby lineage specification could be orchestrated would substantially advance regenerative cell therapies. Yamanaka96 first demonstrated the propensity for four genetic transcription factors (octamer-binding protein 3 (OCT3; also known as OCT4), SOX2, MYC and Krüppellike factor 4 (KLF4)) to induce pluripotency in murine somatic cells, and considerable recent efforts have focused on discovering small-molecule substitutes for these transcription factors to make the overall process more efficient and avoid eventual carcinogenesis. When combined with two or more of these specific genetic factors, small-molecule inhibitors of HDACs, PKMTs or lysine demethylases improve the reprogramming efficiency to a level that is comparable to transduction with all four factors. This demonstrates the key role of epigenetic regulation in cellular reprogramming. For example, valproic acid enables the reprogramming of primary human fibroblasts with two factors, OCT4 and SOX2, without the need for the oncogenes MYC or KLF4. Induced pluripotent stem cells created under these conditions resemble human embyronic stem cells in pluripotency, global gene expression profiles and epigenetic states97. Similarly, the G9A inhibitor BIX-01294 improved reprogramming efficiency in neural progenitor cells transduced with only OCT3/OCT4 and KLF4 (REF. 98). These studies suggest that the generation of induced pluripotent stem cells using only small molecules may soon become feasible. Drugging the epigenome There is experimental evidence for small-molecule inhibition of each of the major classes of acetyl and methyl readers, writers and erasers (FIGS 3,5), although only HDAC inhibitors are currently in the clinic (TABLE 2). The past decade has seen a large increase in the amount of knowledge related to the biochemistry, substrate selectivity and three-dimensional structures of these classes of proteins, revealing common structural and mechanistic features of their active sites. This knowledge has enabled the recent reports of new inhibitors for HATs, histone methyltransferases, lysine methyltransferases, bromodomains and malignant brain tumour domains (MBT domains), which are discussed below. HDACs. HDACs are divided into five phylogenetic classes99 (FIG. 2): class I comprises HDAC1, HDAC2, HDAC3 and HDAC8; class IIa comprises HDAC4, HDAC5, HDAC7 and HDAC9; class IIb comprises HDAC6 and HDAC10; class III comprises the sirtuins SIRT1–SIRT7; and class IV contains HDAC11. Enzymes from classes I, II and IV REVIEWS NATURE REVIEWS | DRUG DISCOVERY VOLUME 11 | MAY 2012 | 391 © 2012 Macmillan Publishers Limited. All rights reserved

REVIEWS Table HDAC and sirtuin inhibitors in clinical development Target Indications Further information Refs Vorinostat Oncology Approved 111,176 Romidepsin Oncology Approved 111,177 Panobinostat Oncology Phasell 111.178 Oncology Phasell 111.179 Entinostat HDAC1 and HDAC2 Phasell 111.180 Mocetinostat HDAC1 and HDAC? Oncology Phase ll 111,181,182 Resminostat HDACLHDAC3 Oncology Phasell Se6a0wgovdenther 183 HDAC(classlandl) Phasell NCT02707 NCT0161624 184 SB939 Pan-HDAC Myelofibrosis Phasell C NC0200 185.186 CUDC-101 6ai9ovuentne 187 PC24781 HDAC (classland Il) Oncology Phasel/ tbi0igovtdente 188 45C-202 HDAC(class) Oncology Phasel 6iegrvdeaie - AR-42 HDAC(classlandl) Oncology Phasel e6aghbgovidenter 189 CG200745 Pan-HDAC Oncology Phasel e6iebg9ordeatice 190 ACY-1215 HDAC6 Oncology Phase l/1l NCTdener EVP-0334 HDAC(classl) Alzheimer's disease Phasel See the EnVivo Pharmaceuticals 191 webs RG2833 HDAC3 Friedreich'sataxia See the RepliGen website ional new drua) SEN196 SIRT1 See the Siena Biotech website 121 divalent metalion for cataynd ADP-ni ically unrelated to the other class soccupy the canonical acetyl lysine channel -histone substrates.For example,HDAC6 is not catalytic site media e selectivity tumour cae in the uch tides,benzamides c nancies.and two dr e first approved for the eat the sulfhyc ate the ca cetylation of both histone but it has been r rted that vorinostat preferentially 392 MAY 2012 VOLUME 11 www.nature.com/reviews/drugdisc 2012 Macmillan Publishers Limited.All riehts reserved
Malignant brain tumour domains Conserved sequence motifs found in certain developmental proteins. These domains bind to mono- or dimethylated lysine-containing peptides and, when deleted in fruitflies, lead to brain tumours. require a divalent metal ion for catalysis100. Sirtuins are NAD+ -dependent enzymes with protein deacetylase and ADP-ribosylase activity, and are structurally and biochemically unrelated to the other classes101,102. Reflecting the ubiquitous distribution of acetyl marks within the cell103, HDACs deacetylate both histone and non-histone substrates. For example, HDAC6 is not involved in epigenetic signalling but it deacetylates microtubules and heat shock protein 90 (REFS 104,105). Several metal-dependent HDAC inhibitors are in the clinic (FIG. 3); most of these target haematological malignancies, and two drugs, vorinostat and romidepsin (Istodax; Celgene), were first approved for the treatment of cutaneous T cell lymphoma in 2006 and 2009, respectively106,107. Increased acetylation of both histone and non-histone substrates mediated by these drugs and related agents is linked to the arrest of tumour cell growth, apoptosis and anti-angiogenesis108,109. All HDAC inhibitors occupy the canonical acetyllysine channel of HDACs (FIG. 4). Interactions at the surface-accessible rim and at a ‘foot pocket’ next to the catalytic site mediate selectivity110, and chelation of a zinc ion at the metal-dependent catalytic site drives both potency and selectivity111. Several types of HDAC inhibitors — such as hydroxamates, cyclic peptides, benzamides and fatty acids — differentially satisfy these pharmacophoric rules. The hydroxamic acid group of vorinostat and the sulfhydryl group of romidepsin chelate the catalytic zinc ion with little specificity between different HDACs, but it has been reported that vorinostat preferentially Table 2 | HDAC and sirtuin inhibitors in clinical development Compound Targets Indications Highest clinical status Further information Refs Vorinostat HDAC1, HDAC2, HDAC3 and HDAC6 Oncology Approved – 111,176 Romidepsin HDAC1, HDAC2, HDAC3 and HDAC8 Oncology Approved – 111,177 Panobinostat HDAC1, HDAC2, HDAC3 and HDAC6 Oncology Phase III ClinicalTrials.gov identifiers: NCT01034163 and NCT01023308 111,178 Belinostat HDAC1, HDAC2, HDAC3 and HDAC6 Oncology Phase II ClinicalTrials.gov identifiers: NCT00873119, NCT00865969, NCT00431340 and others 111,179 Entinostat HDAC1 and HDAC2 Oncology Phase II ClinicalTrials.gov identifiers: NCT00866333, NCT00828854, NCT01349959 and others 111,180 Mocetinostat HDAC1 and HDAC2 Oncology Phase II – 111,181,182 Resminostat HDAC1, HDAC3 and HDAC6 Oncology Phase II ClinicalTrials.gov identifier: NCT01037478 183 Givinostat HDAC (class I and II) Inflammation, oncology Phase II ClinicalTrials.gov identifiers: NCT00928707 and NCT01261624 184 SB939 Pan-HDAC Myelofibrosis Phase II ClinicalTrials.gov identifiers: NCT01112384 and NCT01200498 185,186 CUDC-101 HDACs, EGFR and HER2 Oncology, solid tumours Phase Ib ClinicalTrials.gov identifier: NCT01171924 187 PCI-24781 HDAC (class I and II) Oncology Phase I/II ClinicalTrials.gov identifier: NCT01027910 188 4SC-202 HDAC (class I) Oncology Phase I ClinicalTrials.gov identifier: NCT01344707 – AR-42 HDAC (class I and II) Oncology Phase I ClinicalTrials.gov identifier: NCT01129193 189 CG200745 Pan-HDAC Oncology Phase I ClinicalTrials.gov identifier: NCT01226407 190 ACY-1215 HDAC6 Oncology Phase I/II ClinicalTrials.gov identifier: NCT01323751 – EVP-0334 HDAC (class I) Alzheimer’s disease Phase I See the EnVivo Pharmaceuticals website 191 RG2833 HDAC3 Friedreich’s ataxia Preclinical (investigational new drug) See the RepliGen website – SEN196 SIRT1 Huntington’s disease Phase II See the Siena Biotech website 127 EGFR, epidermal growth factor receptor; HDAC, histone deacetylase; SIRT1, sirtuin 1. REVIEWS 392 | MAY 2012 | VOLUME 11 www.nature.com/reviews/drugdisc © 2012 Macmillan Publishers Limited. All rights reserved
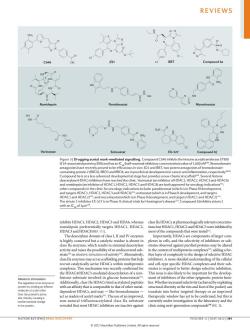
REVIEWS NO: 9 d C646 HN 0 NH HN Entinosta EX-527 re ugging acetyl mark-mediated signalling.Con ound C646 inhibits the hist cts hav BEiwopOtenta C6 d f .HDAC3 and HDAC with anICof1μm inhibits HDACI, HDAC2,HDAC HDACI,HDAC2. most of the compoun is class Ila enzymes,which results in minimal deacetylase strates observed against purified proteins may be altere ors.A more detailed understanding of the cellular he HDAC4/HDAC5-mediated deacetylation ofanon c stimulation Additionally.class na HDACs bind acetvlated peptide lies wheth d selectivity (achie netal- act as readers marksl The e ofan im ved. thera cuticwindowha et to be c non-nat ural triflu under investigation in the oratory and the NATURE REVIEWS DRUG DISCOVERY VOLUME 11 MAY 2012 393
Nature Reviews | Drug Discovery N H N O N N O N Cl N H H N OH O HN NH HN HO O O N N N NH HN NH2 O C646 JQ1 IBET Compound 6a Panobinostat Vorinostat Mocetinostat O N N Cl S N N O O N O OH O N N O N H O HN O NH2 H N H2N O Cl OH O NH N S Romidepsin Entinostat EX-527 Compound 6J HN HN O O O NH O O S S HN O NO2 S N O O O N Allosteric stimulation The regulation of an enzyme or protein by binding an effector molecule at a site other than the protein’s active site, thereby causing a conformational change in the protein. inhibits HDAC1, HDAC2, HDAC3 and HDA6, whereas romidepsin preferentially targets HDAC1, HDAC2, HDAC3 and HDAC8 (REF. 111). The deacetylase domain of class I, II and IV enzymes is highly conserved but a catalytic residue is absent in class IIa enzymes, which results in minimal deacetylase activity and raises the possibility of an undiscovered substrate112 or allosteric stimulation of activity113. Alternatively, class IIa enzymes may act as scaffolding proteins that help recruit catalytically active HDACs within multiprotein complexes. This mechanism was recently confirmed for the HDAC4/HDAC5-mediated deacetylation of a nonhistone substrate involved in glucose homeostasis114. Additionally, class IIa HDACs bind acetylated peptides with an affinity that is comparable to that of other metaldependent HDACs, and may — like bromodomains — act as readers of acetyl marks111. The use of an improved, non-natural trifluoroacetylated class IIa substrate revealed that most HDAC inhibitors are inactive against class IIa HDACs at pharmacologically relevant concentrations but HDAC1, HDAC2 and HDAC3 were inhibited by most of the compounds that were tested111. Importantly, HDACs are components of larger complexes in cells, and the selectivity of inhibitors or substrates observed against purified proteins may be altered in the context of multiprotein complexes115, adding a further layer of complexity to the design of selective HDAC inhibitors. A more detailed understanding of the cellular and cell-type specific HDAC complexes and their substrates is required to better design selective inhibition. This issue is also likely to be important for the development of inhibitors of the other epigenetic protein families. Whether increased selectivity (achieved by exploiting structural diversity at the rim and foot of the pocket) can translate into better targeted therapy or an improved therapeutic window has yet to be confirmed, but this is currently under investigation in the laboratory and the clinic using next-generation compounds108 (FIG. 3). Figure 3 | Drugging acetyl mark-mediated signalling. Compound C646 inhibits the histone acetyltransferase EP300 (E1A-associated protein p300) and has an IC50 (half-maximal inhibitory concentration) value of 1,600 nM133. Bromodomain antagonists have recently proved to be efficacious in vivo: JQ1 and IBET, two potent antagonists of bromodomaincontaining protein 2 (BRD2), BRD3 and BRD4, are in preclinical development in cancer and inflammation, respectively36,76. Compound 6a is at a less advanced developmental stage but provides a new chemical scaffold174. Several histone deacetylase (HDAC) inhibitors have reached the clinic. Vorinostat (an inhibitor of HDAC1, HDAC2, HDAC3 and HDAC6) and romidepsin (an inhibitor of HDAC1, HDAC2, HDAC3 and HDAC8) are both approved for oncology indications111; other compounds in the clinic for oncology indications include: panobinostat (which is in Phase III development, and targets HDAC1, HDAC2, HDAC3 and HDAC6)111; entinostat (which is in Phase II development, and targets HDAC1 and HDAC2)111; and mocetinostat (which is in Phase II development, and targets HDAC1 and HDAC2) 111. The sirtuin 1 inhibitor EX‑527 is in Phase II clinical trials for Huntington’s disease127. Compound 6J inhibits sirtuin 2 with an IC50 of 1μm175. REVIEWS NATURE REVIEWS | DRUG DISCOVERY VOLUME 11 | MAY 2012 | 393 © 2012 Macmillan Publishers Limited. All rights reserved
按次数下载不扣除下载券;
注册用户24小时内重复下载只扣除一次;
顺序:VIP每日次数-->可用次数-->下载券;
- 《药物化学》课程文献资料(Medicinal Chemistry)Application of the Three-Dimensional Structures of Protein Target Molecules in Structure-Based Drug Design.pdf
- 《药物化学》课程文献资料(Medicinal Chemistry)Structural Mechanism for Statin Inhibition of HMG-CoA Reductase.pdf
- 《药物化学》课程文献资料(Medicinal Chemistry)Structural basis for inhibition of a voltage-gated Ca2+ channel by Ca2+ antagonist drugs.pdf
- 《药物化学》课程文献资料(Medicinal Chemistry)Prioritization of cancer therapeutic targets using CRISPR–Cas9 screens.pdf
- 《药物化学》课程文献资料(Medicinal Chemistry)Discovery of a selective inhibitor of oncogenic B-Raf kinase with potent antimelanoma activity.pdf
- 《药物化学》课程文献资料(Medicinal Chemistry)Clinical efficacy of a RAF inhibitor needs broad target blockade in BRAF-mutant melanoma.pdf
- 《药物化学》课程文献资料(Medicinal Chemistry)Name of common cyclic compounds.docx
- 中国科学技术大学:《药物化学》课程教学资源(PPT课件讲稿)Chapter 5 癌症 Cancer(2/2).ppt
- 中国科学技术大学:《药物化学》课程教学资源(PPT课件讲稿)Chapter 7 作用神经系统的药物(中枢神经系统 CNS).ppt
- 中国科学技术大学:《药物化学》课程教学资源(PPT课件讲稿)Chapter 5 癌症 Cancer(1/2).ppt
- 中国科学技术大学:《药物化学》课程教学资源(PPT课件讲稿)Chapter 6 心血管药物 Cardiovascular Drugs.ppt
- 中国科学技术大学:《药物化学》课程教学资源(PPT课件讲稿)Chapter 4 药物的构效关系(SAR).ppt
- 中国科学技术大学:《药物化学》课程教学资源(PPT课件讲稿)Chapter 3b 前体药物 Prodrug.ppt
- 中国科学技术大学:《药物化学》课程教学资源(PPT课件讲稿)Chapter 3a 药代动力学PKPD(DMPK).ppt
- 中国科学技术大学:《药物化学》课程教学资源(PPT课件讲稿)Chapter 2b 先导化合物优化 Lead Optimization.ppt
- 中国科学技术大学:《药物化学》课程教学资源(PPT课件讲稿)Chapter 2a Drug screening & discovery(design).ppt
- 中国科学技术大学:《药物化学》课程教学资源(PPT课件讲稿)Chapter 1 Introduction Medicinal Chemistry(授课教师:阮科).ppt
- 《物理化学》课程教学资源:物理化学 Physical Chemistry(简明版)各章习题与答案(第二版,共十章,天津大学物理化学教研室编).pdf
- 《物理化学》课程教学资源:物理化学 Physical Chemistry(简明版)教材PDF电子版(第二版,共十章,天津大学物理化学教研室编).pdf
- 清华大学出版社:《物理化学》课程书籍教材(上下册)PDF电子版(上下册共十二章,编著:朱文涛).pdf
- 《药物化学》课程文献资料(Medicinal Chemistry)GLIVEC(STI571, IMATINIB),A RATIONALLY DEVELOPED,TARGETED ANTICANCER DRUG,Nat Rev Drug Disc 2002.pdf
- 《药物化学》课程文献资料(Medicinal Chemistry)Personalized medicine in oncology - the future is now.pdf
- 《药物化学》课程文献资料(Medicinal Chemistry)Selective inhibition of BET bromodomains.pdf
- 三明学院:化学工程与工艺专业课程教学大纲汇编.pdf
- 三明学院:材料化学专业课程教学大纲汇编.pdf
- 三明学院:资源与化工学院材料化学专业课程教学大纲汇编.pdf
- 绍兴文理学院:化学化工学院应用化学专业课程教学大纲汇编.pdf
- 绍兴文理学院:化学化工学院化学专业课程教学大纲汇编.pdf
- 绍兴文理学院:化学化工学院高分子材料与工程专业课程教学大纲汇编.pdf
- 单宁化学(书籍文献)Tannin Chemistry.pdf
- 花色苷(书籍文献)Anthocyanins,Kevin Gould · Kevin Davies · Chris Winefield,Biosynthesis, Functions, and Applications.pdf
- 山东第一医科大学(泰山医学院):《基础化学》课程教学资源(教案大纲,打印版)理论教学大纲(负责人:董建).pdf
- 山东第一医科大学(泰山医学院):《基础化学》课程教学资源(教案大纲,打印版)基础化学实验教案.pdf
- 山东第一医科大学(泰山医学院):《基础化学》课程教学资源(习题与解析,打印版)绪论.pdf
- 山东第一医科大学(泰山医学院):《基础化学》课程教学资源(习题与解析,打印版)缓冲溶液.pdf
- 山东第一医科大学(泰山医学院):《基础化学》课程教学资源(习题与解析,打印版)电解质溶液.pdf
- 山东第一医科大学(泰山医学院):《基础化学》课程教学资源(习题与解析,打印版)多相离子平衡.pdf
- 山东第一医科大学(泰山医学院):《基础化学》课程教学资源(习题与解析,打印版)化学反应速率.pdf
- 山东第一医科大学(泰山医学院):《基础化学》课程教学资源(习题与解析,打印版)化学热力学基础.pdf
- 山东第一医科大学(泰山医学院):《基础化学》课程教学资源(习题与解析,打印版)稀溶液的依数性.pdf