《药物化学》课程文献资料(Medicinal Chemistry)Selective inhibition of BET bromodomains

ARTICLE doi:10.1038/mature0950 Selective inhibition of BET bromodomains aagisppkopouuna PicaudYao Shen'Wlm B.m edoroy Elizabeth M.Morse? Christopher A.French,Olaf Wiest Andrew L KungStefan knapp&JamesE.Bradner modules have not yet b ule (JQ that bind by co-crystal main and extra-tern al(BET)family nd provide a versatile chemical Gene regulation is fund. rned by eversible functionin ption elongation facto OP-TEF ble o erpre ting the post-an 学 d ha ffector mo ess th 04 as an in- ing protei ns are of subst the -called N NMC).Functio BRD4-NUT onc in maint ining the char cteristic prolifera d b and prompts terminal squam 1t with peptidic subst nty and Th eotentbrofamily amino-terminal b ergent carboxy-terminal recruitment for the tran tion of d h for targeting ped g the MGI transiti n-tro 44 Bir s02115.U5
ARTICLE doi:10.1038/nature09504 Selective inhibition of BET bromodomains Panagis Filippakopoulos1 *, Jun Qi2 *, Sarah Picaud1 *, Yao Shen3 , William B. Smith2 , Oleg Fedorov1 , Elizabeth M. Morse2 , Tracey Keates1 , Tyler T. Hickman4 , Ildiko Felletar1 , Martin Philpott1 , Shonagh Munro5 , Michael R. McKeown2,6, Yuchuan Wang7 , Amanda L. Christie8 , Nathan West2 , Michael J. Cameron4 , Brian Schwartz4 , Tom D. Heightman1 , Nicholas La Thangue5 , Christopher A. French4 , Olaf Wiest3 , Andrew L. Kung8,9, Stefan Knapp1,5 & James E. Bradner2,6 Epigenetic proteins are intently pursued targets in ligand discovery. So far, successful efforts have been limited to chromatin modifying enzymes, or so-called epigenetic ‘writers’ and ‘erasers’. Potent inhibitors of histone binding modules have not yet been described. Here we report a cell-permeable small molecule (JQ1) that binds competitively to acetyl-lysine recognition motifs, or bromodomains. High potency and specificity towards a subset of human bromodomains is explained by co-crystal structures with bromodomain and extra-terminal (BET) family member BRD4, revealing excellent shape complementarity with the acetyl-lysine binding cavity. Recurrent translocation of BRD4 is observed in a genetically-defined, incurable subtype of human squamous carcinoma. Competitive binding by JQ1 displaces the BRD4 fusion oncoprotein from chromatin, prompting squamous differentiation and specific antiproliferative effects in BRD4-dependent cell lines and patient-derived xenograft models. These data establish proof-of-concept for targeting protein–protein interactions of epigenetic ‘readers’, and provide a versatile chemical scaffold for the development of chemical probes more broadly throughout the bromodomain family. Gene regulation is fundamentally governed by reversible, non-covalent assembly of macromolecules1 . Signal transduction to RNA polymerase requires higher-ordered protein complexes, spatially regulated by assembly factors capable of interpreting the post-translational modification states of chromatin2 . Readers of epigenetic marks are structurally diverse proteins each possessing one or more evolutionarily conserved effector modules, which recognize covalent modifications of histone proteins or DNA. The e-N-acetylation of lysine residues (Kac) on histone tails is associated with an open chromatin architecture and transcriptional activation3 . Context-specific molecular recognition of acetyl-lysine is principally mediated by bromodomains. Bromodomain-containing proteins are of substantial biological interest, as components of transcription factor complexes and determinants of epigenetic memory4 . There are 41 diverse human proteins containing a total of 57 bromodomains. Despite large sequence variations, all bromodomain modules share a conserved fold comprising a left-handed bundle of four a helices (aZ, aA, aB, aC), linked by diverse loop regions (ZA and BC loops) that contribute to substrate specificity. Co-crystal structures with peptidic substrates showed that the acetyl-lysine is recognized by a central hydrophobic cavity and is anchored by a hydrogen bond with an asparagine residue present in most bromodomains5 . The BET family (BRD2, BRD3, BRD4 and BRDT) shares a common domain architecture featuring two amino-terminal bromodomains that exhibit high levels of sequence conservation, and a more divergent carboxy-terminal recruitment domain (Supplementary Fig. 1)6 . Recent research has established a compelling rationale for targeting BRD4 in cancer. BRD4 remains bound to transcriptional start sites of genes expressed during the M/G1 transition, influencing mitotic progression4 . BRD4 is also a critical mediator of transcriptional elongation, functioning to recruit the positive transcription elongation factor complex (P-TEFb)7,8. Cyclin-dependent kinase-9, a core component of P-TEFb9–11, is a validated target in chronic lymphocytic leukaemia12, and has recently been linked to c-Myc-dependent transcription13. Thus, BRD4 recruits P-TEFb to mitotic chromosomes resulting in increased expression of growth-promoting genes14. Importantly, BRD4 has recently been identified as a component of a recurrent t(15;19) chromosomal translocation in an aggressive form of human squamous carcinoma15,16. Such translocations express the tandem N-terminal bromodomains of BRD4 as an in-frame chimaera with the NUT (nuclear protein in testis) protein, genetically defining the so-called NUT midline carcinoma (NMC). Functional studies in patient-derived NMC cell lines have validated the essential role of the BRD4–NUT oncoprotein in maintaining the characteristic proliferation advantage and differentiation block of this uniformly fatal malignancy17. Notably, RNA silencing of BRD4–NUT arrests proliferation and prompts terminal squamous differentiation. These observations underscore the broad utility and immediate therapeutic potential of a direct-acting inhibitor of human bromodomain proteins. A selective and potent inhibitor of BET family bromodomains A major collaborativefocus of our research groups concerns the development of chemical probes18,19 and the optimization of therapeutic leads for the translation of small-molecule modulators of epigenetic targets as cancer therapeutics. Motivated by the above rationale, we have developed biochemical platforms for the identification of new inhibitors of bromodomain isoforms using high-throughput screening, as well as the annotation of putative ligands emerging from collaborative and published research. In the course of these studies, we learned of an *These authors contributed equally to this work. 1 Department of Clinical Medicine, Structural Genomics Consortium, University of Oxford, Old Road Campus, Roosevelt Drive, Oxford OX3 7DQ, UK. 2 Department of Medical Oncology, Dana-Farber Cancer Institute, Harvard Medical School, 44 Binney Street, Boston, Massachusetts 02115, USA. 3 Walther Cancer Research Center and Department of Chemistry and Biochemistry, University of Notre Dame, Notre Dame, Indiana 46556, USA. 4 Department of Pathology, Brigham & Women’s Hospital, Harvard Medical School, 75 Francis Street, Boston, Massachusetts 02115, USA. 5 Department of Clinical Pharmacology, University of Oxford, Old Road Campus, Roosevelt Drive, Oxford OX3 7DQ, UK. 6 Department of Medicine, Harvard Medical School, 25 Shattuck Street, Boston, Massachusetts 02115, USA. 7 Department of Imaging, Dana-Farber Cancer Institute, Harvard Medical School, 44 Binney Street, Boston, Massachusetts 02115, USA. 8 Lurie Family Imaging Center, Dana-Farber Cancer Institute, Harvard Medical School, 44 Binney Street, Boston, Massachusetts 02115, USA. 9 Department of Pediatric Oncology, Dana-Farber Cancer Institute and Children’s Hospital, Boston, Harvard Medical School, 44 Binney Street, Boston, Massachusetts 02115, USA. 23/30 DECEMBER 2010 | VOL 468 | NATURE | 1067 ©2010 Macmillan Publishers Limited. All rights reserved
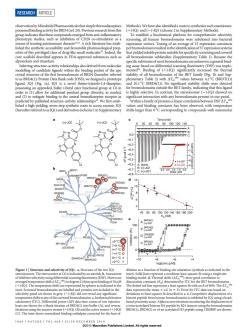
RESEARCH ARTICLE observation by Mitsubishi Pha Methods).We have also identified a route to synthesize each enantiome ous re this nall human bromodomains were n svs nd triazola s within the b nding po the the toas BRD4(D):Protein Data Bank code2 ned Table )withT igand,JQ1 (Fig.la 10.1 BRD4(I to (1)all gnly se ctive, ne ster -JQ1 showe ished struc Within a f mily of proteins orrelation bet en DSF AT referedtoasjQlandac 020406080700120140 :8 0品品。 d12 ERD42 -8 nts a least twith an Rof The grey.(-)-JQ1 c e into buffer (A)an smonitorigg B()or of an BBP are showr 1o6INATUREIVoL46sI2i/B0DEceSI2nPbhsLnd.Arosmenad
observation byMitsubishi Pharmaceuticals that simple thienodiazepines possessed binding activityfor BRD4 (ref. 20). Previous researchfrom this group indicates that these compounds emerged from anti-inflammatory phenotypic studies, such as inhibition of CD28 co-stimulation as a means of treating autoimmune diseases21,22. A rich literature has established the synthetic accessibility and favourable pharmacological properties of this privileged class of drug-like small molecules23. Indeed, the core scaffold described appears in FDA-approved substances such as alprazolam and triazolam. Inferring structure–activity relationships also derived from molecular modelling of candidate ligands within the binding pocket of the apo crystal structure of the first bromodomain of BRD4 (hereafter referred to as BRD4(1); Protein Data Bank code 2OSS), we designed a prototype ligand, JQ1 (Fig. 1a). JQ1 is a novel thieno-triazolo-1,4-diazepine, possessing an appended, bulky t-butyl ester functional group at C6 in order to (1) allow for additional pendant group diversity, as needed, and (2) to mitigate binding to the central benzodiazepine receptor as predicted by published structure–activity relationships23. We first established a high-yielding, seven-step synthetic route to access racemic JQ1 (hereafter referred to as JQ1) and derivatives (scheme 1 in Supplementary Methods).We have also identified a route to synthesize each enantiomer, (1)-JQ1 and (2)-JQ1 (scheme 2 in Supplementary Methods). To establish a biochemical platform for comprehensive selectivity screening, all human bromodomains were subcloned into bacterial expression vectors. Testing of an average of 15 expression constructs per bromodomain resulted in the identification of 37 expression systems that yielded soluble protein suitablefor specificity screening and covered all bromodomain subfamilies (Supplementary Table 1). Because the specific substrates of most bromodomains are unknown, a general binding assay based on differential scanning fluorimetry (DSF) was implemented24. Binding of (1)-JQ1 significantly increased the thermal stability of all bromodomains of the BET family (Fig. 1b and Supplementary Table 2) with DTm obs values between 4.2 uC (BRDT(1)) and 10.1 uC (BRD4(1)). No significant stability shifts were detected for bromodomains outside the BET family, indicating that this ligand is highly selective. In contrast, the stereoisomer (2)-JQ1 showed no significant interaction with any bromodomain present in our panel. Within a family of proteins a linear correlation between DSFDTm obs values and binding constants has been observed, with temperature shifts larger than 6 uC corresponding to compounds with nanomolar log[conc.(μM)] Inhibition (%) –10 –8 –6 –4 0 50 100 BRD4(1) (+)-JQ1 BRD4(2) (+)-JQ1 CREBBP (+)-JQ1 BRD4(1) (–)-JQ1 40 60 80 100 120 140 160 180 200 4 6 8 10 12 ΔT o m bs (°C) Kd (nM) 0 20 40 60 80 100 120 140 –0.8 –0.7 –0.6 –0.5 –0.4 –0.3 –0.2 –0.1 0.0 Time (min) ΔP (μcal s–1) 0.0 0.5 1.0 1.5 2.0 Molar ratio –8 –6 –4 –2 kcal mol–1 of injectant 0 A B C (–)-JQ1 A: blank (+)-JQ1 B: (–)-JQ1 C: (+)-JQ1 >9 °C 7–9 °C 4–7 °C 1–4 °C 0–1 °C <0 °C CREBBP EP300 BAZ1A BRD8(1) BRD8(2) BRDT(1) BRD4(1) BRD2(1) BRD3(1) BRDT(2) BRD3(2) BRD2(2) BRD4(2) BRPF1 BRPF3 BRD9 BRD7 KIAA1240 ATAD2 WDR9(2) PHIP(2) BRWD3(2) BAZ1B BAZ2B BAZ2A ZMYND11 PRKCBP1 TAF1(1) TAF1L(1) TAF1(2) TAF1L(2) CECR2 FALZ GCN5L2 PCAF WDR9(1) BRWD3(1) PHIP(1) ASH1L PB1(3) PB1(6) PB1(1) PB1(4) PB1(5) PB1(2) SMARCA2 SMARCA4 MLL SP110 SP100 SP140 TRIM28 TIF1 TRIM66 TRIM33 LOC93349 BRD1 (+)-JQ1 (–)-JQ1 Cl N N O O S N N Cl N N O O S N N a c b d e Figure 1 | Structure and selectivity of JQ1. a, Structure of the two JQ1 stereoisomers. The stereocentre at C6 is indicated by an asterisk. b, Assessment of inhibitor selectivity using differential scanning fluorimetry (DSF). Shown are averaged temperature shifts (DTm obs) in degrees Celsius upon binding of 10 mM (1)-JQ1. The temperature shifts are represented by spheres as indicated in the inset. Screened bromodomains are labelled and proteins not included in the selectivity panel are shown in grey. (2)-JQ1 did not reveal any significant temperature shifts to any of the screened bromodomains. c, Isothermal titration calorimetry (ITC). Differential power (DP) data time course of raw injection heats are shown for a blank titration of BRD4(1) into buffer (A), and reverse titrations using the inactive isomer (2)-JQ1 (B) and the active isomer (1)-JQ1 (C). The inset shows normalized binding enthalpies corrected for the heat of dilution as a function of binding site saturation (symbols as indicated in the inset). Solid lines represent a nonlinear least squares fit using a single-site binding model. d, Thermal shifts (DTm obs) show good correlation to dissociation constants (Kd) determined by ITC for the BET bromodomains. The dotted red line represents a least squares fit with an R of 96%. The DTm obs data represent the mean 6 s.d. (n 5 3). Error for ITC data was based on deviations to least squares fit described in c. e, Competitive displacement of a histone peptide from human bromodomains is exhibited by JQ1 using a beadbased proximity assay. Alpha-screen titrations monitoring the displacement of a tetra-acetylated histone H4 peptide by JQ1 isomers using the bromodomains BRD4(1), BRD4(2) or of an acetylated H3 peptide using CREBBP are shown. RESEARCH ARTICLE 1068 | NATURE | VOL 468 | 23/30 DECEMBER 2010 ©2010 Macmillan Publishers Limited. All rights reserved
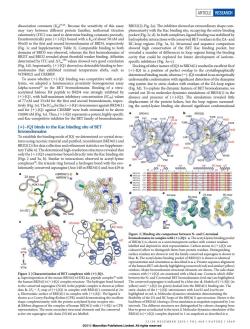
ARTICLE RESEARCH D2(2):Fi T ty with Fig. hle hin he nun lues sho ed very go correlation cavity that could be explored for future development ofisinding R9(2)and CRI ding mode,w hereas()-Q1 resul ed ir with assa ring em due to steric s of the ZA-loop region lated his H4 P peptide to BRD4 was stro f BET of BRD4()in the ce and mce of (+)-JQ1. The ation revealed litt :1 Y139 (nds to the Kac bindingsite of BET Toestablish the binding mode of determined co-crystal stru and b).Si in a lutionarily conserved asparagine (Asn 140 in BRD40D)and Asn 429n and C-termina ms in (+)-JQI a t of BRD2(2)is shown in id lina.c.F onof BET with (+)-JQ Q1 ar of( (+)-1O1.Ths li ement with the p 2010 Mac
dissociation constants (Kd) 25,26. Because the sensitivity of this assay may vary between different protein families, isothermal titration calorimetry (ITC) was used to determine binding constants precisely. Enantiomerically pure (1)-JQ1 bound with a Kd of about 50 nM and 90 nM to the first and second bromodomains of BRD4, respectively (Fig. 1c and Supplementary Table 3). Comparable binding to both domains of BRD3 was observed, whereas the first bromodomains of BRDT and BRD2 revealed about threefold weaker binding. Affinities determined by ITC and DTm obs values showed very good correlation (Fig. 1d). Importantly, (1)-JQ1 showed no detectable binding to bromodomains that exhibited minimal temperature shifts, such as WDR9(2) and CREBBP. To assess whether (1)-JQ1 binding was competitive with acetyllysine, we adapted a luminescence proximity homogeneous assay (alpha-screen)27 to the BET bromodomains. Binding of a tetraacetylated histone H4 peptide to BRD4 was strongly inhibited by (1)-JQ1, with half-maximum inhibitory concentration (IC50) values of 77 nM and 33 nM for the first and second bromodomain, respectively (Fig. 1e). The IC50 for the (2)-JQ1 stereoisomer against BRD4(1) and for (1)-JQ1 against CREBBP were both estimated to be above 10,000 nM (Fig. 1e). Thus, (1)-JQ1 represents a potent, highly specific and Kac-competitive inhibitor for the BET family of bromodomains. (1)-JQ1 binds to the Kac binding site of BET bromodomains To establish the binding mode of JQ1 we determined co-crystal structures using racemic material and purified, recombinant BRD4(1) and BRD2(2) (for data collection and refinement statistics see Supplementary Table 4). The determined high-resolution structures revealed that only the (1)-JQ1 enantiomer bound directly into the Kac binding site (Figs 2 and 3a, b). Similar to interactions observed in acetyl-lysine complexes28, the triazole ring formed a hydrogen bond with the evolutionarily conserved asparagine (Asn 140 in BRD4(1) and Asn 429 in BRD2(2); Fig. 2a). The inhibitor showed an extraordinary shape complementarity with the Kac binding site, occupying the entire binding pocket (Fig. 2c, d). In both complexes, ligand binding was stabilized by hydrophobic interactions with conserved BET residues in the ZA- and BC-loop regions (Fig. 3a, b). Structural and sequence comparison showed high conservation of the BET Kac binding pocket, but revealed a number of differences in loop regions lining the binding cavity that could be explored for future development of isoformspecific inhibitors (Fig. 3a–c). Docking of either isomer of JQ1 to BRD4(1) resulted in excellentfit of (1)-JQ1 in a position of perfect overlap to the crystallographically determined binding mode, whereas (2)-JQ1 resulted in an energetically unfavourable conformation with significant distortion of the diazepine ring system due to steric clashes with residues of the ZA-loop region (Fig. 3d). To explore the dynamic features of BET bromodomains, we carried out 20-ns molecular dynamics simulations of BRD4(1) in the absence and presence of (1)-JQ1. The simulations revealed little displacement of the protein helices, but the loop regions surrounding the acetyl-lysine binding site showed significant conformational αC αZ αA ZA loop BC loop N140 αB –10 kT/e +10 kT/e mBRD4(1) hBRD4(1) (+)-JQ1 H3K14ac N140 ZA loop BC loop a b c d Figure 2 | Characterization of BET complexes with (1)-JQ1. a, Superimposition of the mouse BRD4(1)–H3K14ac peptide complex28 with the human BRD4(1)–(1)-JQ1 complex structure. The hydrogen bond formed to the conserved asparagine (N140) in the peptide complex is shown as yellow dots. b, 2Fo 2 Fc map of (1)-JQ1 in complex with BRD4(1) contoured at 2s. c, Electrostatic surface of BRD4(1) in complex with (1)-JQ1. The ligand is shown as a Corey–Pauling–Koltun (CPK) model demonstrating the excellent shape complementarity with the protein acetylated lysine receptor site. d, Ribbon diagram of the complex of human BRD4(1) with (1)-JQ1 in CPK representation. The main secondary structural elements and the conserved active site asparagine side chain (N140) are labelled. ...|....|....|....|....|....|....|....|....|....|....|....|....|....|... BRD2(1) 97 WPFRQPVDAVKLGLPDYHKIIKQPMDMGTIKRRLENNYYWAASECMQDFNTMFTNCYIYNKPTDDIVLMAQT BRD2(2) 370 WPFYKPVDASALGLHDYHDIIKHPMDLSTVKRKMENRDYRDAQEFAADVRLMFSNCYKYNPPDHDVVAMARK BRD3(1) 57 WPFYQPVDAIKLNLPDYHKIIKNPMDMGTIKKRLENNYYWSASECMQDFNTMFTNCYIYNKPTDDIVLMAQA BRD3(2) 332 WPFYKPVDAEALELHDYHDIIKHPMDLSTVKRKMDGREYPDAQGFAADVRLMFSNCYKYNPPDHEVVAMARK BRD4(1) 81 WPFQQPVDAVKLNLPDYYKIIKTPMDMGTIKKRLENNYYWNAQECIQDFNTMFTNCYIYNKPGDDIVLMAEA BRD4(2) 374 WPFYKPVDVEALGLHDYCDIIKHPMDMSTIKSKLEAREYRDAQEFGADVRLMFSNCYKYNPPDHEVVAMARK BRDT(1) 50 WPFQRPVDAVKLQLPDYYTIIKNPMDLNTIKKRLENKYYAKASECIEDFNTMFSNCYLYNKPGDDIVLMAQA BRDT(2) 293 WPFYNPVDVNALGLHNYYDVVKNPMDLGTIKEKMDNQEYKDAYKFAADVRLMFMNCYKYNPPDHEVVTMARM ZA loop αA αB BC loop αC ZA loop BC loop BC loop ZA loop L92 L94 0 (ns) 20 C425 M438 D434 V435 H433 N429 L383 Y386 L381 V376 K374 W370 P371 Y428 L92 M149 D145 D144 I146 C136 Y97 Y139 N140 V87 Q85 W81 P82 L94 a b c BRD4(1) BRD2(2) de f Figure 3 | Binding site comparison between N- and C-terminal bromodomains in complex with (1)-JQ1. a, The acetyl-lysine binding pocket of BRD4(1) is shown as a semi-transparent surface with contact residues labelled and depicted in stick representation. Carbon atoms in (1)-JQ1 are coloured yellow to distinguish them from protein residues. Distinguishing surface residues are shown in red; the family conserved asparagine is shown in blue. b, The acetyl-lysine binding pocket of BRD2(2) is shown in identical representation and orientation as described in a. c, Protein sequence alignment of the human BET sub-family highlighting conserved (red) and similar (yellow) residues. Major bromodomain structural elements are shown. The side-chain contacts with (1)-JQ1 are annotated with a black star. Contacts which differ between the N- and C-terminal BET bromodomains (red star) are highlighted. The conserved asparagine is indicated by a blue star. d, Models of (1)-JQ1 (in yellow) and (2)-JQ1 (in green) docked into the BRD4(1) binding site. The steric clashes of the (2)-JQ1 stereoisomer with Leu 92 and Leu 94 are highlighted in red. e, Molecular dynamics simulation demonstrating the flexibility of the ZA and BC loops of the BRD4(1) apo-structure. Shown is the backbone of BRD4(1) during a 20-ns simulation as snapshots separated by 1-ns intervals. The different structures are distinguished by colours changing from blue to green as indicated in the inset. f, Molecular dynamics simulation of the BRD4(1)–(1)-JQ1 complex depicted in 1-ns snapshots as described in e. ARTICLE RESEARCH 23/30 DECEMBER 2010 | VOL 468 | NATURE | 1069 ©2010 Macmillan Publishers Limited. All rights reserved
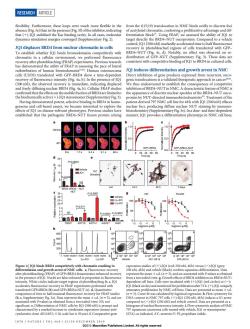
RESEARCH ARTICLE flexibility.Furtherr from the t(15:19)translocation in NMC binds avidly to discrete foci T onc protein to a vehic JQl displaces BRD4 from nuclear chromatin in cells BRD4-N TGEP-NUT (S hing(FRAP) ious redistribution of human bro JQl induces differentiation and growth arrest in NMC cells (U2 ow a tim M). the observe o es ons bile fraction of BrD4are of discrete nuclea ckles of the BRD4-NUT o hemically active 1g r48 d to exp the foci,pro NU g by immu established that the path fusion protein arising manner,JQl pro a differentiation type in NMC cell lines 89℃ 001 P0.01 .0001P<0.0001 0 911010 aeO1bn杰BRD photob re pre A y of NMC cells by Q (50M 1070I NATURE I VOL 468 1 23/30 DECEMBE 2010 ⊥.All rights reserved
flexibility. Furthermore, these loops were much more flexible in the absence (Fig. 3e) than in the presence (Fig. 3f) of the inhibitor, indicating that (1)-JQ1 stabilized the Kac binding cavity. In all cases, molecular dynamics simulation energies converged (Supplementary Fig. 2). JQ1 displaces BRD4 from nuclear chromatin in cells To establish whether JQ1 binds bromodomains competitively with chromatin in a cellular environment, we performed fluorescence recovery after photobleaching (FRAP) experiments. Previous research has demonstrated the utility of FRAP in assessing the pace of lateral redistribution of human bromodomains17,29. Human osteosarcoma cells (U2OS) transfected with GFP–BRD4 show a time-dependent recovery of fluorescence intensity (Fig. 4a, b). In the presence of JQ1 (500 nM), the observed recovery is immediate, indicating displaced and freely diffusing nuclear BRD4 (Fig. 4a, b). Cellular FRAP studies confirmed that the effects on the mobilefraction of BRD4 are limited to the biochemically active (1)-JQ1 stereoisomer (Supplementary Fig. 3). Having demonstrated potent, selective binding to BRD4 in homogeneous and cell-based assays, we became interested to explore the effects of JQ1 on disease-relevant phenotypes. Previous studies have established that the pathogenic BRD4–NUT fusion protein arising from the t(15;19) translocation in NMC binds avidly to discrete foci of acetylated chromatin, conferring a proliferative advantage and differentiation block17. Using FRAP, we assessed the ability of JQ1 to target directly the BRD4–NUT oncoprotein. Compared to a vehicle control, JQ1 (500 nM) markedly accelerated time to half fluorescence recovery in photobleached regions of cells transfected with GFP– BRD4–NUT (Fig. 4c, d). Notably, no effect was observed on redistribution of GFP–NUT (Supplementary Fig. 3). These data are consistent with competitive binding of JQ1 to BRD4 in cultured cells. JQ1 induces differentiation and growth arrest in NMC Direct inhibition of gene products expressed from recurrent, oncogenic translocations is a validated therapeutic approach in cancer30,31. We thus endeavoured to establish the consequences of competitive inhibition of BRD4–NUT in NMC. A characteristic feature of NMC is the appearance of discrete nuclear speckles of the BRD4–NUT oncoprotein by NUT-directed immunohistochemistry32. Treatment of the patient-derived 797 NMC cell line for 48 h with JQ1 (500 nM) effaces nuclear foci, producing diffuse nuclear NUT staining by immunohistochemistry (Supplementary Fig. 3e). In a dose- and time-dependent manner, JQ1 provokes a differentiation phenotype in NMC cell lines, Vehicle JQ1 Pre 1.7 s 5.4 s 9.1 s 60 s 0 20 40 60 80 0.0 0.5 1.0 Time (s) Normalized intensity 0 20 40 60 80 0.0 0.5 1.0 Time (s) Normalized intensity GFP–BRD4 GFP–BRD4–NUT a b c 0 1 2 3 4 20 25 30 35 TGM1 KRT10 KRT14 RAD21 RAN Relative expression P=0.011 NS P<0.0001 P<0.0001 P<0.0001 797 (Keratin) t=0 24 h 48 h 7 days Vehicle (+)-JQ1 (–)-JQ1 d f g P=0.020 P=0.0049 NS BRD4 NUT 0 5 10 15 20 Vehicle JQ1 Recovery t1/2 (s) BRD4– NUT e 797 –3 –2 –1 0 1 0 50 100 log[conc.(μM)] –3 –2 –1 0 1 log[conc.(μM)] Proliferation Per403 JQ1 (48 h) 12 43 45 STA (48 h) 8 55 37 Count 0 800 600 200 400 0 100,000 50,000 0 100,000 50,000 0 100,000 50,000 Veh. (–)-JQ1 (+)-JQ1 105 0 104 103 102 105 104 103 102 105 0 104 103 102 105 0 104 103 102 0 105 104 103 102 0 105 104 103 102 0 Vehicle 3.2 86 9.7 AV PI h i 0 50 100 Proliferation Figure 4 | JQ1 binds BRD4 competitively with chromatin resulting in differentiation and growth arrest of NMC cells. a, Fluorescence recovery after photobleaching (FRAP) of GFP–BRD4 demonstrates enhanced recovery in the presence of JQ1. Nuclei are false-coloured in proportion to fluorescence intensity. White circles indicate target regions of photobleaching. b, c, JQ1 accelerates fluorescence recovery in FRAP experiments performed with transfected GFP–BRD4 (b) and GFP–BRD4–NUT (c). d, Quantitative comparison of time to half-maximal fluorescence recovery for FRAP studies (b, c, Supplementary Fig. 3a). Data represent the mean 6 s.d. (n 5 5), and are annotated with P-values as obtained from a two-tailed t-test. NS, not significant. e, Differentiation of NMC cells by JQ1 (500 nM) is prompt and characterized by a marked increase in cytokeratin expression (mouse anticytokeratin clone AE1/AE3; 310, scale bar is 50 mm). f, Comparative gene expression studies of (1)-JQ1 (red; 250 nM, 48 h) versus (2)-JQ1 (grey; 250 nM, 48 h) and vehicle (black) confirm squamous differentiation. Data represent the mean 6 s.d. (n 5 3), and are annotated with P-values as obtained from a two-tailed t-test. g, Growth effects of BRD4 inhibition on BRD4–NUTdependent cell lines. Cells were incubated with (1)-JQ1 (red circles) or (2)- JQ1 (black circles) and monitored for proliferation after 72 h. (1)-JQ1 uniquely attenuates proliferation by NMC cell lines. Data are presented as mean 6 s.d. (n 5 3). Curve fit was calculated by logistical regression. h, Flow cytometry for DNA content in NMC 797 cells. (1)-JQ1 (250 nM, 48 h) induces a G1 arrest compared to (2)-JQ1 (250 nM) and vehicle control. Data are presented as a histogram of nuclear fluorescence intensity. i, Flow cytometric analysis of NMC 797 squamous carcinoma cells treated with vehicle, JQ1 or staurosporine (STA), as indicated. AV, annexin-V; PI, propidium iodide. RESEARCH ARTICLE 1070 | NATURE | VOL 468 | 23/30 DECEMBER 2010 ©2010 Macmillan Publishers Limited. All rights reserved
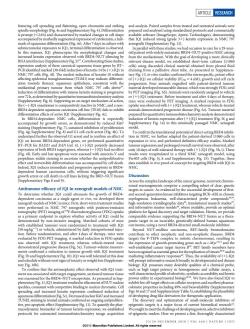
ARTICLE RESEARCH cell nd analysis.Paired s and untreated animal prparestandardized protocols and commercially y ma ous differe el evant disease arkedt-tonductora iot in ds thor the tumo is by PET in aging (Fi (-JQ1 treatr d).Animals wererando vehicl 2h,as and al days of t uptake w vehie-re 5 of ac JQ1 (Supp mentary Fig.4c) aredforguantathreimmnohistoeh stry analysis demonstrate cas de BRD4 inhibi- ct o 1060 AN (r)( and late ar ed with ar nov and day ed,induces immediate and pr poptosis in BRD4 NMC Discussion (Supplementary Figs 8 and9) Across the complex landscape of the cancer genome,chromo cy of JQl in xenogr It models s of NMC enograft models st,short- rm treatment studie d info ailabl Herein,we pro id re rar sof the with JQl treatm vchicle-reated mice at this r weigh tary Fig.10b) mical probe 16 uclear Ki67 and ine ivatives for thera he ing drugp apeutic app -3 lemen cq 20 gh VEEMBE2010IVOL ATORE1071
featuring cell spreading and flattening, open chromatin and striking spindle morphology (Fig. 4e and Supplementary Fig. 4). Differentiation is prompt (,24 h) and characterized by marked changes in cell shape accompanied by markedly augmented expression of cytokeratin, a hallmark of squamous differentiation (Fig. 4e). After 7 days in culture with submicromolar exposures to JQ1, terminal differentiation is observed. In this manner, JQ1 phenocopies the morphological changes and increased keratin expression observed with BRD4–NUT silencing by RNAinterference (Supplementary Fig. 5)17. Corroborating these studies, expression analysis of three canonical squamous tissue genes by RT– PCR identified marked (30-fold) induction of keratin-14 by (1)-JQ1 in NMC 797 cells (Fig. 4f). The modest induction of keratin-10 without affecting epidermal transglutaminase (TGM1) may indicate differentiation towards thoracic squamous epithelium, consistent with the mediastinal primary tumour from which NMC 797 cells derive33. Induction of differentiation with intense keratin staining is progressive over 72 h, as determined by quantitative immunohistochemistry analysis (Supplementary Fig. 6). Supporting an on-target mechanism-of-action, the (2)-JQ1 enantiomer is comparatively inactive in NMC, and a nonBRD4-dependent squamous carcinoma cell line (TE10) fails to exhibit differentiation effects of active JQ1 (Supplementary Fig. 4c). In BRD4-dependent NMC cells, differentiation is expectedly accompanied by growth arrest, as demonstrated by reduced Ki67 staining (Supplementary Fig. 7), sustained inhibition of proliferation (Fig. 4g; Supplementary Fig. 8) and G1 cell-cycle arrest (Fig. 4h). To understand further the observed G1 arrest and to confirm an effect of JQ1 on known BRD4-dependent genes, we performed quantitative RT–PCR for RAD21 and RAN (ref. 4). (1)-JQ1 potently decreased expression of both BRD4 target genes, whereas (2)-JQ1 had no effect (Fig. 4f). Early and late apoptosis were assessed with annexin-V and propidium iodide staining to ascertain whether the antiproliferative effect and irreversible differentiation was accompanied by cell death. Indeed, JQ1 induces immediate and progressive apoptosis in BRD4- dependent human carcinoma cells, without triggering significant growth arrest or cell death in cell lines lacking the BRD–NUT fusion (Supplementary Figs 8 and 9). Antitumour efficacy of JQ1 in xenograft models of NMC To determine whether JQ1 could attenuate the growth of BRD4- dependent carcinoma as a single agent in vivo, we developed three xenograft models of NMC in mice. First, short-term treatment studies were performed in NMC 797 xenografts with positron-emission tomography (PET) imaging of 18F-fluorodeoxyglucose (FDG) uptake as a primary endpoint to explore whether activity of JQ1 could be demonstrated by non-invasive imaging. Matched cohorts of mice with established tumours were randomized to treatment with JQ1 (50 mg kg21 ) or vehicle, administered by daily intraperitoneal injection. Before randomization, and after 4 days of therapy, mice were evaluated by FDG-PET imaging. A marked reduction in FDG uptake was observed with JQ1 treatment, whereas vehicle-treated mice demonstrated progressive disease (Fig. 5a). Tumour-volume measurements confirmed a reduction in tumour growth with JQ1 treatment (Fig. 5b and Supplementary Fig. 10). JQ1 was well tolerated at this dose and schedule without overt signs of toxicity or weight loss (Supplementary Fig. 10b). To confirm that the antineoplastic effect observed with JQ1 treatment was associated with target engagement, sectioned tumour tissue was examined for the BRD4–NUT oncoprotein. As presented in Supplementary Fig. 11, JQ1 treatment resulted in effacement of NUT nuclear speckles, consistent with competitive binding to nuclear chromatin. Cell spreading and increased keratin expression confirmed induction of squamous differentiation (Fig. 5c). Decreased nuclearKi67 andincreased TUNEL staining in treated animals confirmed an ongoing antiproliferative, pro-apoptotic effect (Supplementary Fig. 11). To quantify the pharmacodynamic biomarker of tumour keratin expression, we established protocols for automated immunohistochemistry image acquisition and analysis. Paired samples from treated and untreated animals were prepared and analysed using standardized protocols and commercially available software (ImageScope; Aperio Technologies), demonstrating that JQ1 induced strong (grade 31) keratin expression in NMC 797 xenografts (Supplementary Fig. 12). In parallel with these studies, we had occasion to care for a 29-yearold patient with widely metastatic BRD4–NUT-positive NMC arising from the mediastinum. With the goal of developing a more clinically relevant disease model, we established short-term cultures (11060 cells) using discarded clinical material obtained from pleural fluid draining from a palliative chest tube. As presented in Supplementary Fig. 13, in vitro studies confirmed the stereospecific, potent effect of (1)-JQ1 on cellular viability (IC50 5 4 nM), growth and cell cycle progression. Four animals engrafted with patient-derived tumour material developed measurable disease, which was strongly FDG-avid by PET imaging (Fig. 5d). Animals were randomly assigned to vehicle or (1)-JQ1 treatment. Before treatment and after 4 days of therapy, mice were evaluated by PET imaging. A marked response in FDG uptake was observed with (1)-JQ1 treatment, whereas vehicle-treated animals demonstrated progressive disease (Fig. 5e). Tumour material preparedfor quantitative immunohistochemistry analysis demonstrated induction of keratin expression after (1)-JQ1 treatment (Fig. 5f, g and Supplementary Fig. 14) in this minimally passaged NMC xenograft model. To confirm the translational potential of direct-acting BRD4 inhibition in NMC, we further adapted the patient-derived 11060 cells to expansion in vivo, and performed definitive efficacy studies. Marked tumour regression and prolonged overall survival were observed, after only 18 days of well-tolerated therapy with (1)-JQ1 (Fig. 5h, i). These results were recapitulated in a third NMC xenograft model, using Per403 cells (Fig. 5j, k and Supplementary Fig. 15). Together, these data establish in vivo proof-of-concept for targeting BRD4 with JQ1 in NMC. Discussion Across the complex landscape of the cancer genome, recurrent chromosomal rearrangements comprise a compelling subset of clear, genetic targets in cancer. As evidenced by the successful development of firstand second-generation kinase inhibitors targeting BCR–ABL in chronic myelogenous leukaemia, well-characterized probe compounds34,35, high-resolution crystallographic data36, translational research studies37, and informative murine models38, where available, provide an optimal platform for ligand discovery and target validation. Herein, we provide comparable evidence supporting the BRD4–NUT fusion as a therapeutic target in an incurable, genetically-defined human squamous carcinoma, using a novel BRD4-directed small-molecule inhibitor. Beyond NUT-midline carcinoma, BET-family bromodomains contribute to other neoplastic and non-neoplastic diseases. BRD4 targets the P-TEFb complex to mitotic chromosomes, resulting in the expression of growth-promoting genes such as c-Myc12,14 and the well-established cancer target Aurora B39. BET family members have been recognized as essential genesfor the replication of viruses40,41 and in mediating inflammatory responses42. Thus, the availability of (1)-JQ1 will prompt informative research broadly in developmental and disease biology. JQ1 possesses many desirable qualities of a chemical probe, such as high target potency in homogeneous and cellular assays, a well-characterized profile of selectivity, synthetic accessibility and herein proven utility in experimental biology18,19. We have also found JQ1 to exhibit few off-target effects on cellular receptors and excellent pharmacokinetic properties including 49% oral bioavailability (Supplementary Figs 16 and 17 and Supplementary Table 5), establishing the plausibility of developing drug-like derivatives for therapeutic application. The discovery and optimization of small-molecule inhibitors of epigenetic targets is a major focus of current biomedical research43. We sought to meet the challenge of developing potent, selective inhibitors of epigenetic readers. Here we present a first, thoroughly characterized ARTICLE RESEARCH 23/30 DECEMBER 2010 | VOL 468 | NATURE | 1071 ©2010 Macmillan Publishers Limited. All rights reserved

RESEARCH ARTICLE 2,000 500- 000 P<0.000 1.000 500 ging of m EH ath of prin 20 d in s d (n=7) 97 urs e from ant antitat asks are n (mo +)-IOI (red har is 2 MC ival (i,k)in p t-derived 1100(h)and Per3 m0 10 in all groups)Asg 0) 11060 fts to (+)-01 (50mgko erallsurvival by a log-rank test (00001)Black c asdemonstrated by PET hicle. inhibitor of the beT-family of bromodomains.The a into th readers for ligand discovery. flank of 6 METHODS SUMMARY gri an Women's Hospital itches signal transduction and urce beamline X10SA. igaku FR-E 1.Ptashne,M 15.209) d by 2骑 ein.B. 3.M 73 391(9 4 10721NArURE1voL46i12130DEcE%2 n Publishers Limited.All rights reserved
inhibitor of the BET-family of bromodomains. The approach outlined herein further establishes the feasibility of abrogating protein–protein interactions with small molecules, and targeting additional epigenetic readers for ligand discovery. METHODS SUMMARY The inhibitor JQ1 was synthesized in both racemic and enantiomerically pure format using the synthetic route outlined in scheme 1 and scheme 2 (Supplementary Methods) and its structure was fully characterized. Human bromodomains were expressed in bacteria as His-tagged proteins and were purified by nickelaffinity and gel-filtration chromatography. Protein integrity was assessed by SDS–PAGE and electro-spray mass spectrometry on an Agilent 1100 Series LC/MSD TOF. All crystallizations were carried out at 4 uC using the sitting-drop vapour-diffusion method. X-ray diffraction data were collected at the Swiss Light source beamline X10SA, or using a Rigaku FR-E generator. Structures were determined by molecular replacement. Isothermal titration calorimetry experiments were performed at 15 uC on a VP-ITC titration microcalorimeter (MicroCal). Thermal melting experiments were carried out on an Mx3005p RT–PCR machine (Stratagene) using SYPRO Orange as a fluorescence probe. Dose-ranging small-molecule studies of proliferation were performed in white, 384-well plates (Corning) in DMEM media containing 10% FBS. Compounds were delivered with a JANUS pin-transfer robot and proliferation measurements were made on an Envisionmultilabel plate-reader (PerkinElmer).Murine xenografts were established by injecting NMC cells in 30% Matrigel (BD Biosciences) into the flank of 6-week-old female NCr nude mice (Charles River Laboratories). Tumour measurements were assessed by caliper measurements, and volume was calculated using the formula Vol 5 0.53 L3W2 . All mice were humanely killed, and tumours were fixed in 10% formalin for histopathological examination. Quantitative immunohistochemistry was performed using the Aperio Digital Pathology Environment (Aperio Technologies) at the DF/HCC Core Laboratory at the Brigham and Women’s Hospital. Received 5 May; accepted 17 September 2010. Published online 24 September 2010. 1. Ptashne, M. Binding reactions: epigenetic switches, signal transduction and cancer. Curr. Biol. 19, R234–R241 (2009). 2. Schreiber, S. L. & Bernstein, B. E. Signaling network model of chromatin. Cell 111, 771–778 (2002). 3. Marushige, K. Activation of chromatin by acetylation of histone side chains. Proc. Natl Acad. Sci. USA 73, 3937–3941 (1976). 4. Dey, A., Nishiyama, A., Karpova, T., McNally, J. & Ozato, K. Brd4 marks select genes on mitotic chromatin and directs postmitotic transcription. Mol. Biol. Cell 20, 4899–4909 (2009). a d JQ1 Vehicle Keratin Ki67 b Vehicle JQ1 Pre Post 0 500 1,000 1,500 2,000 Vehicle JQ1 Tumour volume (mm3) P = 0.039 Vehicle JQ1 5 10 15 20 % ID per g e Pre Post P = 0.0001 JQ1 Veh. Keratin Mask Vehicle JQ1 0 20 40 60 80 100 Positivity (%) c f g 0 10 20 30 0 1,000 2,000 3,000 Day of treatment 0 10 20 30 Day of treatment 0 10 20 30 Day of treatment 0 10 20 30 Day of treatment Tumour volume (mm3) 0 50 100 Per cent survival Tumour volume (mm3) Per cent survival 0 500 1,000 1,500 2,000 0 50 100 P < 0.0001 P < 0.0001 h i j k P < 0.0001 P < 0.0001 Figure 5 | JQ1 promotes differentiation, tumour regression and prolonged survival in murine models of NMC. a, PET imaging of murine NMC 797 xenografts. FDG uptake in xenograft tumours is reduced by 50 mg kg21 JQ1 treatment compared to vehicle control. Arrows indicate the anatomical location of tumour xenograft. b, Tumour volume is reduced in mice with established disease (NMC 797 xenografts) treated daily with 50 mg kg21 JQ1 compared to vehicle control. A significant response to therapy is observed by a two-tailed t-test at 14 days (P 5 0.039). Data represent the mean 6 s.d. (n 5 7). c, Histopathological analysis of NMC 797 tumours excised from animals treated with JQ1 reveals induction of keratin expression (mouse anticytokeratin clone AE1/AE3, 340) and impaired proliferation (Ki67, 340), as compared to vehicle-treated animals (scale bar is 20 mm). d, Viability of patientderived NMC 11060 xenografts was confirmed by PET imaging. Arrow indicates the anatomical location of tumour xenograft. e, Therapeutic response of primary 11060 NMC xenografts to (1)-JQ1 (50 mg kg21 daily for 4 days) was demonstrated by PET imaging. Integrated signal encompassed within the tumour volume is presented as the per cent injected dose per gram (% ID per g). f, Histopathological analysis of primary NMC 11060 tumours excised from animals treated with (1)-JQ1 reveals induction of keratin expression (mouse anti-cytokeratin clone AE1/AE3,320; scale bar is 20 mm), compared to vehicletreated animals. Quantitative analysis of keratin induction was performed using image masking (f, right panel) and pixel positivity analysis (g). A significant response to therapy is observed by a two-tailed t-test (P 5 0.0001). Data represent the mean 6 s.d. of three independent wide microscopic fields. Comparative images of stained excised tumours and quantitative masks are provided in Supplementary Fig. 14. h–k, (1)-JQ1 (red circles and lines; 50 mg kg21 daily for 18 days) produces a decrease in tumour volume (h, j) and promotes prolonged survival (i, k) in patient-derived 11060 (h, i) and Per403 (j, k) NMC xenograft models (n 5 10 in all groups). A significant response to therapy is observed for tumour volume by a two-tailed t-test (P , 0.0001) and for overall survival by a log-rank test (P , 0.0001). Black circles and lines, vehicle. RESEARCH ARTICLE 1072 | NATURE | VOL 468 | 23/30 DECEMBER 2010 ©2010 Macmillan Publishers Limited. All rights reserved

ARTICLE RESEARCH 33.Toretsky,⊥.A.etal.Tra ts Am nal5a9apco8ng 26080620 6 34 ransd y by a protei cation and intran 9 ⊥.2hu 002) ntyrosne cyclin subunits 12.755 3> e kinase on the 39.0 295035103(200 n by the bromodomain protein Brd4 You.etal K pdb6aaraaiceoc 41 ional pause release Cel/141432-445 4 082 5g时ao 2006 s for histone-moditying enzymes.Nature Chem.BioL 4 a nove on is linked to the online version of the paper a nia5,4414720o9 nd A.Bass 0/2006/129623)2001 e th signed JOl and es ic routes. S.and (PM-1) 27. ed prol 28 ccess 30. treatment of acut ssions i s hrad Ld.v
5. Owen, D. J. et al.The structural basis for the recognition of acetylated histone H4 by the bromodomain of histone acetyltransferase gcn5p. EMBO J. 19, 6141–6149 (2000). 6. Zeng, L. & Zhou, M. M. Bromodomain: an acetyl-lysine binding domain. FEBS Lett. 513, 124–128 (2002). 7. Yang, X. J. Multisite protein modification and intramolecular signaling. Oncogene 24, 1653–1662 (2005). 8. Yang, Z. et al. Recruitment of P-TEFb for stimulation of transcriptional elongation by the bromodomain protein Brd4. Mol. Cell 19, 535–545 (2005). 9. Peng, J., Zhu, Y., Milton, J. T. & Price, D. H. Identification of multiple cyclin subunits of human P-TEFb. Genes Dev. 12, 755–762 (1998). 10. Marshall, N. F. & Price, D. H. Purification of P-TEFb, a transcription factor required for the transition into productive elongation. J. Biol. Chem. 270, 12335–12338 (1995). 11. Marshall, N. F., Peng, J., Xie, Z. & Price, D. H. Control of RNA polymerase II elongation potential by a novel carboxyl-terminal domain kinase. J. Biol. Chem. 271, 27176–27183 (1996). 12. Phelps, M. A. et al. Clinical response and pharmacokinetics from a phase 1 study of an active dosing schedule of flavopiridol in relapsed chronic lymphocytic leukemia. Blood 113, 2637–2645 (2009). 13. Rahl, P. B. et al. c-Myc regulates transcriptional pause release. Cell 141, 432–445 (2010). 14. Yang, Z., He, N. & Zhou, Q. Brd4 recruits P-TEFb to chromosomes at late mitosis to promote G1 gene expression and cell cycle progression. Mol. Cell. Biol. 28, 967–976 (2008). 15. French, C. A. et al. BRD4 bromodomain gene rearrangement in aggressive carcinoma with translocation t(15;19). Am. J. Pathol. 159, 1987–1992 (2001). 16. French, C. A. et al. BRD4-NUT fusion oncogene: a novel mechanism in aggressive carcinoma. Cancer Res. 63, 304–307 (2003). 17. French, C. A. et al. BRD-NUT oncoproteins: a family of closely related nuclear proteins that block epithelial differentiation and maintain the growth of carcinoma cells. Oncogene 27, 2237–2242 (2008). 18. Frye, S. V. The art of the chemical probe. Nature Chem. Biol. 6, 159–161 (2010). 19. Oprea, T. I. et al. A crowdsourcing evaluation of the NIH chemical probes. Nature Chem. Biol. 5, 441–447 (2009). 20. Miyoshi, S., Ooike, S., Iwata, K., Hikawa, H. & Sugaraha, K. Antitumor agent. International Patent No. PCT/JP2008/073864 (WO/2009/084693) (2009). 21. Adachi, K. et al. Thienotriazolodiazepine compound and a medicinal use thereof. International Patent No. PCT/JP2006/310709 (WO/2006/129623) (2006). 22. Sueoka, H., Komatsu, H., Kobayashi, H. & Ehara, S. Thienotriazolodiazepine Compounds and Medicinal Uses Thereof 1–50 (Yoshitomi Pharmaceutical Industries, Ltd, 1998). 23. VonVoigtlander, P. F. & Straw, R. N. Alprazolam: Review of pharmacological, pharmacokinetic and clinical data. Drug Dev. Res. 6, 1–12 (1985). 24. Niesen, F. H., Berglund, H. & Vedadi, M. The use of differential scanning fluorimetry to detect ligand interactions that promote protein stability. Nature Protocols 2, 2212–2221 (2007). 25. Fedorov, O. et al. A systematic interaction map of validated kinase inhibitors with Ser/Thr kinases. Proc. Natl Acad. Sci. USA 104, 20523–20528 (2007). 26. Bullock, A. N. et al. Structural basis of inhibitor specificity of the human protooncogene proviral insertion site in moloney murine leukemia virus (PIM-1) kinase. J. Med. Chem. 48, 7604–7614 (2005). 27. Quinn, A. M. et al. A homogeneous method for investigation of methylationdependent protein-protein interactions in epigenetics. Nucleic Acids Res. 38, e11 (2010). 28. Vollmuth, F., Blankenfeldt, W. & Geyer, M. Structures of the dual bromodomains of the P-TEFb-activating protein Brd4 at atomic resolution. J. Biol. Chem. 284, 36547–36556 (2009). 29. Dey, A. et al.A bromodomain protein, MCAP, associates with mitotic chromosomes and affects G2-to-M transition. Mol. Cell. Biol. 20, 6537–6549 (2000). 30. Huang, M. E. et al. Use of all-trans retinoic acid in the treatment of acute promyelocytic leukemia. Blood 72, 567–572 (1988). 31. Druker, B. J. et al. Efficacy and safety of a specific inhibitor of the BCR-ABL tyrosine kinase in chronic myeloid leukemia. N. Engl. J. Med. 344, 1031–1037 (2001). 32. Haack, H. et al. Diagnosis of NUT midline carcinoma using a NUT-specific monoclonal antibody. Am. J. Surg. Pathol. 33, 984–991 (2009). 33. Toretsky, J. A. et al. Translocation (11;15;19): a highly specific chromosome rearrangement associated with poorly differentiated thymic carcinoma in young patients. Am. J. Clin. Oncol. 26, 300–306 (2003). 34. Buchdunger, E. et al. Selective inhibition of the platelet-derived growth factor signal transduction pathway by a protein-tyrosine kinase inhibitor of the 2-phenylaminopyrimidine class. Proc. Natl Acad. Sci. USA 92, 2558–2562 (1995). 35. Buchdunger, E. et al. Inhibition of the Abl protein-tyrosine kinase in vitro and in vivo by a 2-phenylaminopyrimidine derivative. Cancer Res. 56, 100–104 (1996). 36. Schindler, T. et al. Structural mechanism for STI-571 inhibition of abelson tyrosine kinase. Science 289, 1938–1942 (2000). 37. Druker, B. J. et al. Effects of a selective inhibitor of the Abl tyrosine kinase on the growth of Bcr-Abl positive cells. Nature Med. 2, 561–566 (1996). 38. le Coutre, P. et al. In vivo eradication of human BCR/ABL-positive leukemia cells with an ABL kinase inhibitor. J. Natl Cancer Inst. 91, 163–168 (1999). 39. You, J. et al. Regulation of aurora B expression by the bromodomain protein Brd4. Mol. Cell. Biol. 29, 5094–5103 (2009). 40. You, J. et al. Kaposi’s sarcoma-associated herpesvirus latency-associated nuclear antigen interacts with bromodomain protein Brd4 on host mitotic chromosomes. J. Virol. 80, 8909–8919 (2006). 41. Abbate, E. A., Voitenleitner, C. & Botchan, M. R. Structure of the papillomavirus DNA-tethering complex E2:Brd4 and a peptide that ablates HPV chromosomal association. Mol. Cell 24, 877–889 (2006). 42. Huang, B., Yang, X. D., Zhou, M. M., Ozato, K. & Chen, L. F. Brd4 coactivates transcriptional activation of NF-kB via specific binding to acetylated RelA. Mol. Cell. Biol. 29, 1375–1387 (2009). 43. Cole, P. A. Chemical probes for histone-modifying enzymes. Nature Chem. Biol. 4, 590–597 (2008). Supplementary Information is linked to the online version of the paper at www.nature.com/nature. Acknowledgements We are grateful to U. Oppermann, S. Mu¨ller, S. Sallan, C. Lathan, P. Rahl, R. Young, K. Lee and K. Shaw for discussions and sharing unpublished information; K. Agu, S. Johnston and L. Li for analytical chemistry support; J. Daley for flow cytometry support; T. Bowman, T. Caron, C. Marvin and S. Rodig for immunohistochemistry; and A. Bass for sharing cell lines. The Structural Genomics Consortium is a registered charity (number 1097737) that receives funds from the Canadian Institutes for Health Research, the Canadian Foundation for Innovation, Genome Canada through the Ontario Genomics Institute, GlaxoSmithKline, Karolinska Institutet, the Knut and Alice Wallenberg Foundation, the Ontario Innovation Trust, the Ontario Ministry for Research and Innovation, Merck & Co., Inc., the Novartis Research Foundation, the Swedish Agency for Innovation Systems, the Swedish Foundation for Strategic Research and the Wellcome Trust. This research was supported by a Graduate Fellowship from the Chemistry-Biochemistry-Biology Interface Program at the University of Notre Dame, NIGMS T32-075762 (to Y.S.), the DF/HCC (to C.A.F. and J.E.B.), the National Institutes of Health, the Burroughs Wellcome Fund, and the Leukemia & Lymphoma Society (to J.E.B.). Author Contributions P.F., J.Q., S.K. and J.E.B. designed the study, analysed data and wrote the manuscript. P.F. and S.P. performed and analysed biophysical studies. J.Q. and J.E.B. designed JQ1 and established the synthetic routes. Y.S. and O.W. completed docking and molecular dynamics studies. O.F. performed and analysed DSF. S.M. and N.L.T. contributed biochemical assays.M.R.M.,M.P. and T.D.H. performed and analysed alpha-screen assays. W.B.S., M.J.C. and J.E.B. performed in vitro NMC studies and immunohistochemistry. E.M.M. performed flow cytometry studies. E.M.M. and N.W. performed proliferation studies. T.T.H.,M.J.C., C.A.F. and J.E.B. completed FRAP studies. M.R.M. and B.S. performed expression analysis. Y.W., A.L.C. and A.L.K. completed in vivo efficacy studies. T.K. and I.F. expressed and purified proteins. S.K. and J.E.B. supervised the research. Author Information Atomic coordinates and structure factors for the reported crystal structures have been deposited with the Protein Data Bank under accession codes 2OSS (BRD4(1)), 3MXF (BRD4(1)–(1)-JQ1) and 3ONI (BRD2(2)–(1)-JQ1). Reprints and permissions information is available at www.nature.com/reprints. The authors declare no competing financial interests. Readers are welcome to comment on the online version of this article at www.nature.com/nature. Correspondence and requests for materials should be addressed to S.K. (stefan.knapp@sgc.ox.ac.uk) or J.E.B. (james_bradner@dfci.harvard.edu). ARTICLE RESEARCH 23/30 DECEMBER 2010 | VOL 468 | NATURE | 1073 ©2010 Macmillan Publishers Limited. All rights reserved
按次数下载不扣除下载券;
注册用户24小时内重复下载只扣除一次;
顺序:VIP每日次数-->可用次数-->下载券;
- 《药物化学》课程文献资料(Medicinal Chemistry)Personalized medicine in oncology - the future is now.pdf
- 《药物化学》课程文献资料(Medicinal Chemistry)GLIVEC(STI571, IMATINIB),A RATIONALLY DEVELOPED,TARGETED ANTICANCER DRUG,Nat Rev Drug Disc 2002.pdf
- 《药物化学》课程文献资料(Medicinal Chemistry)Epigenetic protein families - a new frontier for drug discovery.pdf
- 《药物化学》课程文献资料(Medicinal Chemistry)Application of the Three-Dimensional Structures of Protein Target Molecules in Structure-Based Drug Design.pdf
- 《药物化学》课程文献资料(Medicinal Chemistry)Structural Mechanism for Statin Inhibition of HMG-CoA Reductase.pdf
- 《药物化学》课程文献资料(Medicinal Chemistry)Structural basis for inhibition of a voltage-gated Ca2+ channel by Ca2+ antagonist drugs.pdf
- 《药物化学》课程文献资料(Medicinal Chemistry)Prioritization of cancer therapeutic targets using CRISPR–Cas9 screens.pdf
- 《药物化学》课程文献资料(Medicinal Chemistry)Discovery of a selective inhibitor of oncogenic B-Raf kinase with potent antimelanoma activity.pdf
- 《药物化学》课程文献资料(Medicinal Chemistry)Clinical efficacy of a RAF inhibitor needs broad target blockade in BRAF-mutant melanoma.pdf
- 《药物化学》课程文献资料(Medicinal Chemistry)Name of common cyclic compounds.docx
- 中国科学技术大学:《药物化学》课程教学资源(PPT课件讲稿)Chapter 5 癌症 Cancer(2/2).ppt
- 中国科学技术大学:《药物化学》课程教学资源(PPT课件讲稿)Chapter 7 作用神经系统的药物(中枢神经系统 CNS).ppt
- 中国科学技术大学:《药物化学》课程教学资源(PPT课件讲稿)Chapter 5 癌症 Cancer(1/2).ppt
- 中国科学技术大学:《药物化学》课程教学资源(PPT课件讲稿)Chapter 6 心血管药物 Cardiovascular Drugs.ppt
- 中国科学技术大学:《药物化学》课程教学资源(PPT课件讲稿)Chapter 4 药物的构效关系(SAR).ppt
- 中国科学技术大学:《药物化学》课程教学资源(PPT课件讲稿)Chapter 3b 前体药物 Prodrug.ppt
- 中国科学技术大学:《药物化学》课程教学资源(PPT课件讲稿)Chapter 3a 药代动力学PKPD(DMPK).ppt
- 中国科学技术大学:《药物化学》课程教学资源(PPT课件讲稿)Chapter 2b 先导化合物优化 Lead Optimization.ppt
- 中国科学技术大学:《药物化学》课程教学资源(PPT课件讲稿)Chapter 2a Drug screening & discovery(design).ppt
- 中国科学技术大学:《药物化学》课程教学资源(PPT课件讲稿)Chapter 1 Introduction Medicinal Chemistry(授课教师:阮科).ppt
- 三明学院:化学工程与工艺专业课程教学大纲汇编.pdf
- 三明学院:材料化学专业课程教学大纲汇编.pdf
- 三明学院:资源与化工学院材料化学专业课程教学大纲汇编.pdf
- 绍兴文理学院:化学化工学院应用化学专业课程教学大纲汇编.pdf
- 绍兴文理学院:化学化工学院化学专业课程教学大纲汇编.pdf
- 绍兴文理学院:化学化工学院高分子材料与工程专业课程教学大纲汇编.pdf
- 单宁化学(书籍文献)Tannin Chemistry.pdf
- 花色苷(书籍文献)Anthocyanins,Kevin Gould · Kevin Davies · Chris Winefield,Biosynthesis, Functions, and Applications.pdf
- 山东第一医科大学(泰山医学院):《基础化学》课程教学资源(教案大纲,打印版)理论教学大纲(负责人:董建).pdf
- 山东第一医科大学(泰山医学院):《基础化学》课程教学资源(教案大纲,打印版)基础化学实验教案.pdf
- 山东第一医科大学(泰山医学院):《基础化学》课程教学资源(习题与解析,打印版)绪论.pdf
- 山东第一医科大学(泰山医学院):《基础化学》课程教学资源(习题与解析,打印版)缓冲溶液.pdf
- 山东第一医科大学(泰山医学院):《基础化学》课程教学资源(习题与解析,打印版)电解质溶液.pdf
- 山东第一医科大学(泰山医学院):《基础化学》课程教学资源(习题与解析,打印版)多相离子平衡.pdf
- 山东第一医科大学(泰山医学院):《基础化学》课程教学资源(习题与解析,打印版)化学反应速率.pdf
- 山东第一医科大学(泰山医学院):《基础化学》课程教学资源(习题与解析,打印版)化学热力学基础.pdf
- 山东第一医科大学(泰山医学院):《基础化学》课程教学资源(习题与解析,打印版)稀溶液的依数性.pdf
- 山东第一医科大学(泰山医学院):《基础化学》课程教学资源(习题与解析,打印版)电极电位.pdf
- 山东第一医科大学(泰山医学院):《基础化学》课程教学资源(习题与解析,打印版)原子结构.pdf
- 山东第一医科大学(泰山医学院):《基础化学》课程教学资源(习题与解析,打印版)分子结构.pdf