甘肃农业大学:食品科学与工程学院(文献讲义)Exploration of activated sludge resistome using metagenomics
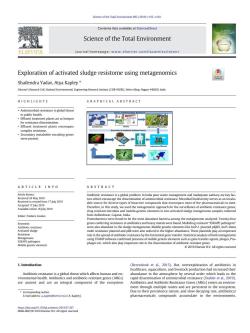
Scerxe of the Total Emvireament 692 (2019)1155-1164 Contents lists available at ScicnceDirect Science of the Total Environment 亚 ELSEVIER journal homepage:www.elsevier.com/locate/scitotenv Exploration of activated sludge resistome using metagenomics Shailendra Yadav.Atya Kapley* e(IR-NEER)Nc HIcHLIGHTs GRAPHICAL ABSTRACT ts act as hotspot olite encoding genes @-+l-g ARTICLE INFO ABSTRACT Article histo 20 m17y201 e for div ds that ost of the pha ticals to dat AC18y209 Editor:Frederic Coulon nd p oticed in t (Berendonk) thcare. are ancient and are an integral component of the ecosystem pharmaceuticals compounds accumulate in the environments sg06v米am
Exploration of activated sludge resistome using metagenomics Shailendra Yadav, Atya Kapley ⁎ Director's Research Cell, National Environmental, Engineering Research Institute (CSIR-NEERI), Nehru Marg, Nagpur 440020, India HIGHLIGHTS • Antimicrobial resistance is global threat to public health. • Effluent treatment plants act as hotspot for resistance dissemination. • Effluent treatment plants encompass complex resistome. • Secondary metabolite encoding genes were present GRAPHICAL ABSTRACT article info abstract Article history: Received 24 May 2019 Received in revised form 17 July 2019 Accepted 17 July 2019 Available online 18 July 2019 Editor: Frederic Coulon Antibiotic resistance is a global problem. In India poor waste management and inadequate sanitary are key factors which encourage the dissemination of antimicrobial resistance. Microbial biodiversity serves as an invaluable source for diverse types of bioactive compounds that encompass most of the pharmaceuticals to date. Therefore, in this study, we used the metagenomic approach for the surveillance of antibiotic resistance genes, drug resistant microbes and mobile-genetic elements in two activated sludge metagenome samples collected from Ankleshwar, Gujarat, India. Proteobacteria were found to be the most abundant bacteria among the metagenome analyzed. Twenty-four genes conferring resistance to antibiotics and heavy metals were found. Multidrug resistant “ESKAPE pathogens” were also abundant in the sludge metagenome. Mobile genetic elements like IncP-1 plasmid pKJK5, IncP-1beta multi resistance plasmid and pB8 were also noticed in the higher abundance. These plasmids play an important role in the spread of antibiotic resistance by the horizontal gene transfer. Statistical analysis of both metagenome using STAMP software confirmed presence of mobile genetic elements such as gene transfer agents, phages, Prophages etc. which also play important role in the dissemination of antibiotic resistant genes. © 2019 Elsevier B.V. All rights reserved Keywords: Antibiotic resistance Activated sludge Resistome Metagenome ESKAPE pathogens Mobile genetic elements 1. Introduction Antibiotic resistance is a global threat which affects human and environmental health. Antibiotics and antibiotic-resistant genes (ARGs) are ancient and are an integral component of the ecosystem (Berendonk et al., 2015). But, overexploitation of antibiotics in healthcare, aquaculture, and livestock production had increased their abundance in the atmosphere by several order which leads to the rapid dissemination of antimicrobial resistance (Stalder et al., 2019). Antibiotics and Antibiotic Resistance Genes (ARGs) enters an environment through multiple routes and are persistent in the ecosystem. Due to their persistence nature, and slow decaying rate, antibiotics/ pharmaceuticals compounds accumulate in the environments. Science of the Total Environment 692 (2019) 1155–1164 ⁎ Corresponding author. E-mail address: a_kapley@neeri.res.in (A. Kapley). https://doi.org/10.1016/j.scitotenv.2019.07.267 0048-9697/© 2019 Elsevier B.V. All rights reserved Contents lists available at ScienceDirect Science of the Total Environment journal homepage: www.elsevier.com/locate/scitotenv

.Yadav.Kapley/Scence of the Total 692(01)1155-1164 as p CeOfanDiotictsgai tro et al 20 dos Santos).The me ic ar nds in the divers tance (Berund.5:Chet 201 201 abun and Antibiotic Res tance Bacteria(ARBs)are ortant factors which pha gonresisto ome profiling of a ctivated sludge from India Mos 2014-Eu e.2017).U5g ted sludge san WWTPs.A ndia ing nex the treat aeC品8a9 mpr ing the a ce of the s,and MGEs in a qured infections(WHO.01).Such first time the study rep orts the al f ARGs and MGE rd to ug re ering antibiotics as a poten 2 Materials methods e (o tl,2017: es et 2.1.Sampling and ype 22013L a it be vska and Pilla.2017 54 ent Plants(STPs ies.Sim PlantE )in higher con tracted fromeach samplng bote in duplicate(1for each samp ance.Also,thes are rich in nutrient concentration,whicl samples w 20181. to like tracted DNA was he WWTPs (A DNA ST pooled togethe wastewat Act tivated sude harbor ore. 22.Preparation of2x150 NextSeq libraries ged as an a ve matter o Metagenomic se 0 DNA HT ing was Truse plat ch Uel 016 L A o ng ource f then c d to bl nds u ng En ng to by 5'to 3'polymerase.Single'A in the past decade and con the a e the 2016 munities (Forbes et al.2017).Metagenomics has the potential to flow cell.The purification of the ligated product was carried out by
Therefore, they are being considered as chemicals of emerging concern or as pollutants. The total amount of resistance gene associated with an ecosystem is known as “resistome” (Finley et al., 2013; Stalder et al., 2019). Generally, antimicrobial resistance develops by natural selection or by adaptation of bacteria in the presence of antibiotics. It is gained either by acquiring resistance genes or by chromosomal mutation. Horizontal Gene Transfer (HGT) plays an essential role in the dissemination of antimicrobial resistance (Berglund, 2015; Che et al., 2019). It accounts for about 75% resistance genes exchange between environments, farm animals, and gut microbiota (Soucy et al., 2015) (von Wintersdorff et al., 2016). Mobile Genetic Elements (MGEs) also acts as a vector for the dissemination of ARGs (Stevenson et al., 2017; Tao et al., 2016). The higher concentration of antibiotics residues, heavy metals and Antibiotic Resistance Bacteria (ARBs) are important factors which contribute in the selection of new ARGs and Multidrug-resistant Bacteria (MRBs) (Andersson and Hughes, 2014; Fluegge, 2017). Usage of antibiotics at the subinhibitory concentration also promotes the wider dissemination of antimicrobial resistance (Andersson and Hughes, 2014). World Health Organization (WHO) has expressed its concern over increasing incidences of resistance, which is compromising the treatment of infectious diseases and causing widespread of communityacquired infections (Hofer, 2019; Willyard, 2017). Mostly, MRBs like Escherichia coli, Klebsiella pneumoniae, and Staphylococcus aureus are responsible for community-acquired infections (WHO, 2014). Such infectious diseases are hard to treat because of the higher drug resistance, which results in a more extended hospital stay and an increase in the treatment cost. Due to the poor sanitary conditions and unregulated use of antibiotics, the problem has become more severe in developing countries (Founou et al., 2017; Holmes et al., 2016). The burden of antibiotic resistance is rising consistently, and India has emerged as the largest consumer of antibiotics (Laxminarayan and Chaudhury, 2016). A significant portion of the Indian population has acquired resistance to antibiotics of one or another type (McKenna, 2013). Thus, it becomes evident that the epidemic of antimicrobial resistance (AMR) is rising globally, with a much higher pace than what has been imagined earlier (Lobanovska and Pilla, 2017). Further, engineered systems like Sewage Treatment Plants (STPs) and Wastewater Treatment Plants (WWTPs) are acting as a reservoir of ARGs (Almakki et al., 2019; Karkman et al., 2017). Because of the prevalence of antibiotic residues, ARBs, and heavy metals at higher concentration, such system acts as a hotspot for the dissemination of resistance. Also, these systems are rich in nutrient concentration, which facilitates cell-cell interaction, thus increasing chances of HGT (Manaia et al., 2018). Antibiotics belonging to classes like β-lactams, fluoroquinolones, tetracyclines, macrolides, etc. have been found in the WWTPs (Almakki et al., 2019; Brunton et al., 2019; Guo et al., 2017; Nnadozie et al., 2017). Activated sludge process is commonly used for biological remediation of pollutants present in wastewater. Activated sludge harbors huge prokaryotic diversity. Bacteria play a key role in the degradation of toxic pollutants (Cai et al., 2016; Kapley et al., 2015). Therefore, the presence of antibiotics and to what extent such compounds can be eliminated by activated sludge process has emerged as an active matter of research (Jelic et al., 2011; Shchegolkova et al., 2016). Microorganisms inhabiting such a complex environment produce secondary metabolites, which may act as a bioresource for the development of novel antimicrobial compounds (Culligan et al., 2014; Lewis et al., 2010). They belong to various chemical classes having antitumor, antiviral, or antibiotic activities (Vaishnav and Demain, 2011). Since no new antibiotic was discovered in the past decade and conventional techniques have failed to yield new antimicrobials, analysis of activated sludge metagenome may increase the chances of discovering novel secondary metabolite with antimicrobial potential (Brown and Wright, 2016; Teitzel, 2019). Metagenomics is the genomic analysis of microbial communities (Forbes et al., 2017). Metagenomics has the potential to discover enormous microbial diversity associated with activated sludge biomass (Jadeja et al., 2014; Lv et al., 2015). Thus, functional screening of metagenomic libraries generated from activated sludge may yield novel biocatalysts having pharmaceutical and commercial importance (Castro et al., 2014; dos Santos et al., 2017). The metagenomic approach is currently used for monitoring the abundance of antibiotic-resistant genes and for the discovery of novel bioactive compounds in the diverse niche (Gatica et al., 2019; He et al., 2019; Lewis, 2017; Nowrotek et al., 2019; Zhang et al., 2019). Reddy & Dubey et al. (2019) used metagenomic approach for the mining of antibiotic and metal ion resistance genes in the water of river Ganga. They found that beta-lactam was most abundant ARGs in the water of Ganga (Reddy and Dubey, 2019). While going through the literature, no articles were found emphasizing on resistome profiling of activated sludge from India. Most of the study available aims on catabolic and taxonomic profiling, targeting the bioremediation potential of microbial consortia (Jadeja et al., 2019; Raina et al., 2019; Sen and Mukhopadhyay, 2019). Therefore, in the present study, two activated sludge samples obtained from WWTPs, Ankleshwar, Gujarat, India were sequenced using nextgeneration Illumina sequencing. The study emphasized on the monitoring the abundance of the ARBs, ARGs, and MGEs in activated sludge metagenome because of their role in the dissemination of AMRs. The present study also investigated the prevalence of biosynthetic gene cluster encoding for secondary metabolites in the activated sludge. For the first time, the study reports the abundance of ARGs and MGEs from an activated sludge sample considering antibiotics as a potent chemical pollutant of WWTPs from India. 2. Materials & methods 2.1. Sampling and qualitative and quantitative analysis of gDNA Samples were collected from both effluent treatment plants situated in Ankleshwar, Gujarat, India. Sample AKR012_contigs was collected from the Common Effluent Treatment Plant (CETP) in five 500 ml sampling bottle treating wastewater generated from dyes and other chemical industries. Similarly, sample A2_S27_assembly was collected from Effluent Treatment Plant (ETP) in five 500 ml sampling bottle treating wastewater from different small-scale industries. The collected samples were immediately preserved using dry ice. Metagenomic DNA was extracted from each sampling bottle in duplicate (10 for each sample) using Fast DNA Spin Kit for Soil, MP Biomedicals. After extraction metagenomic DNA obtained from both activated sludge samples were checked by agarose gel (1%) electrophoresis. Five microliters (μl) of extracted DNA was loaded and checked for the intact band. The agarose gel was run at 85 V for 50 min. The obtained DNA was also checked for purity (A260/280 ratio) using Nanodrop 8000. Positive DNA samples were pooled together and were then sent for sequencing. The concentration of extracted DNA from each sample was determined using Qubit® 2.0 Fluorometer. 2.2. Preparation of 2 ×150 NextSeq libraries Metagenomic sequencing was performed on Illumina Truseq platform using “Nano DNA HT Library Preparation Kit.” Around 200 ng of the obtained DNA was sheared by Covaris. The resulting fragments were then converted to blunt ends using End Repair Mix. 3′ overhangs were removed by the 3′ to 5′ exonuclease, and the subsequent gaps of 5′ overhangs were filled by 5′ to 3′ polymerase. Single ‘A’ nucleotide was added to the 3′ end of blunt fragments to prevent self-ligation during the adapter ligation reaction (Kapley et al., 2015). Single ‘T’ nucleotide was added on the 3′ end of the adapter during its ligation to the fragment to ensure lower chimera or concatenated templates formation. For hybridization, indexing adapters were ligated to the ends of the template DNA fragments and subsequently introduced onto the flow cell. The purification of the ligated product was carried out by 1156 S. Yadav, A. Kapley / Science of the Total Environment 692 (2019) 1155–1164

S.Yadry.A Kapley Science of the Tota r622019)1155-1164 Table 1 nic datasets obtained sludge onle using A2_$27_assembly AKR012_contigs resistance genes are represented as points of larger size and dark color 3.Result and discussion 11502 ldvcyegardigthebateri e m). a5 embly)comprise of bacteri alyze the amplified library ir mprising676%and 8 respectively ir n the two metagenomes.A b (up to s rel drug eema ir resistance(G 20wa20 ples and Enter tive clea a,201 2.4.Metagenomic analysis using MG RAST derived)infection. The two r for such patients.Recently it h hee 0s78554 with D embly)respe uitceresi e against Carbapenem "the last resort antibiotics SAMN03074223(SRA ID:SRR1702227)(A2_S27_ass cstistantbacteri mycin (D and Graham.2017). boicdiversity of the two metagenomes was compared using STAMP done using the Storey q-value (Parks et al.2014).The asse an 32.The occurrence of ARGs and metal resistant genes in activated sludge 60%(Clausen et) 25.Comprehensive analysis of metagenome using BusyBee web-based server for the metag omic analysis(Laczny c2leddistrtbuionofmicrobiomras ciated with two activated sludee sample ched against the genes)using hmmsearch from HMMER(v3.1b2:http://hmmer.janelia
using SP beads supplied in the kit, followed by PCR amplification of sizeselected product as described in the manual (Yadav et al., 2014). High Sensitivity (HS) DNA chip was used to analyze the amplified library in Bioanalyzer 2100 (Agilent Technologies). 2.3. Cluster generation and sequencing After analyzing the library using Bioanalyzer, the positive libraries were loaded onto NextSeq for cluster generation and sequencing. Both the forward and reverse directions NextSeq were performed by implying paired-end sequencing of the template fragments. Binding of samples to complementary adapter oligos was performed by using the kit reagents on the paired-end flow cell. After resynthesis of the reverse strand, the adapters were allowed to bind the selective cleavage of the forward strands, followed by the sequencing from the opposite end of the fragment which was carried out by the copied reverse strand. 2.4. Metagenomic analysis using MG RAST The two metagenome sequences obtained from activated sludge samples were submitted to NCBI with accession number SAMN03074221 (SRA ID: SRS785543) (AKR012_contigs) and SAMN03074223 (SRA ID: SRR1702227) (A2_S27_assembly) respectively. For the analysis of microbial taxonomic abundance and prevalence of antibiotic and metal resistance genes, sequences were analyzed using “MG-RAST” server with default parameter (Keegan et al., 2016). Annotation of metagenomic sequences was given in Table 1. Taxonomic and catabolic diversity was further statically analyzed using Statistical Analysis of Metagenomic Profiles (STAMP) software. A two-sided G test (w/Yates') + Fisher's exact test was implemented for hypothesis testing, whereas the difference in proportions (DPs) and confidence intervals (CIs) for P ¼ 0.95 were calculated using the Newcombe-Wilson method. Multiple test corrections were done using the Storey q-value (Parks et al., 2014). The assembled contigs of both metagenomes were also used for finding acquired antimicrobial resistance genes using ResFinder 3.1 tool of center for genomics epidemiology (CGEwebface@cbs.dtu.dk). The parameter for contigs annotation was threshold id of 90% and a minimum length of 60% (Clausen et al., 2016). 2.5. Comprehensive analysis of metagenome using BusyBee BusyBee is a web-based server for the metagenomic analysis (Laczny et al., 2017). Both taxonomic and functional annotation of metagenome can be performed using BusyBee. It utilizes a bootstrapped approach for the annotation of metagenomic sequences. It requires input files in the fasta format. It utilizes Prokka as a tool for the rapid annotation of microbial genomes (Seemann, 2014). The translated coding sequences are then searched against the ResFams (collection of antibiotic resistance genes) using hmmsearch from HMMER (v3.1b2; http://hmmer.janelia. org/) using hidden Markov models (profile HMMs) (Liu et al., 2016). In BusyBee input sequences are represented as individual points in the 2D scatter plot. Convex hull delineates the predicted cluster. Antibiotic resistance genes are represented as points of larger size and dark color. 3. Result and discussion 3.1. Taxonomic distribution of microbes in activated sludge metagenome Activated sludge samples harbor huge prokaryotic diversity which carries out bioremediation of toxic compounds present in wastewater. Among the microbes, bacterial community plays a major role in the entire process. The two-metagenome obtained from both sludge samples showed remarkably similar microbial diversity regarding the bacterial community. Sample from CETP (AKR012_contigs) and ETP (A2_S27_assembly) comprise of 97.8 and 98.6% of bacteria. Proteobacteria was the predominant among the bacterial community comprising 67.6% and 88.3% respectively in the two metagenomes. Archaea communities were least abundant in both metagenome samples comprising of 0.64 and 0.26%, respectively (Table 2). Taxonomic distribution of microbial diversity has been shown in Fig. 1a and b (up to genus level) and supplementary material (up to phylum level). Recently WHO has released a list of twelve drug-resistant pathogenic bacteria that pose the greatest threat to human health due to their resistance (Göttig et al., 2014; Willyard, 2017). These MRBs, i.e., “Enterococcus spp., Staphylococcus aureus, Klebsiella spp., Acinetobacter baumannii, Pseudomonas aeruginosa, and Enterobacter spp.” are referred as “ESKAPE” pathogens, and they have raised the epidemic of antibiotic resistance by several folds (Lewis et al., 2015). Presence of these bacteria have been recently reported in the Indian subcontinent by various studies and was detected in the activated sludge samples used in the present study (Das et al., 2011; Laxminarayan and Chaudhury, 2016; Walsh et al., 2011). Acinetobacter baumanii is responsible for nosocomial (hospital derived) infection. It causes severe infection to the already critically ill/chronic patients, and no clinical treatment exists for such patients. Recently it has been found that the most strain of Acinetobacter baumanii bacterium has acquired resistance against Carbapenem “the last resort antibiotics” (McKenna, 2013). WHO has categorized Helicobacter pylori as the high-priority drugresistant bacteria, which accounts for more than 95% cases of gastric cancer. Earlier triple therapy was used for the treatment of Helicobacter pylori derived infection, but its efficacy has declined more than 80% from 2000 to 2017 due to the acquisition of resistance against antibiotic Clarithromycin (Dang and Graham, 2017). Enterobacter cloacae were also detected in the sludge metagenome with much abundance, which has been found resistant to colistin antibiotics (Band et al., 2016). Catabolic diversity of the two metagenomes was compared using STAMP software and has been shown in Fig. 2. 3.2. The occurrence of ARGs and metal resistant genes in activated sludge Twenty-four genes conferring resistance to antibiotics and metals were detected in the sludge metagenome using MG-RAST (Fig. 3 a and Table 1 Annotation of metagenomic datasets obtained from two activated sludge sample using MGRAST. Feature A2_S27_assembly AKR012_contigs Total sequences 87,058,937 137,225,975 Total reads 101,935 143,628 Average read length (bases) 854 955 Average GC content 58 ± 13% 62 ± 10% QC passed reads 94,537 123,297 Average read length after QC (bases) 646 ± 705 bp 548 ± 695 bp Predicted protein feature 115,002 144,151 Predicted rRNA features 452 577 Identified protein features 68,545 98,720 Identified functional categories 57,492 85,462 Table 2 Domain level distribution of microbiome associated with two activated sludge sample. Domain A2_S27_assembly (% abundance) AKR012_contigs (% abundance) Bacteria 97.88 98.68 Archaea 0.64 0.25 Eukaryotes 1.02 0.46 Viruses 0.33 0.31 Other 0.14 0.12 S. Yadav, A. Kapley / Science of the Total Environment 692 (2019) 1155–1164 1157

15 a) 4s圆 g1.T hA2.S271s
Fig. 1. Taxonomic diversity (upto genus level) associated with both (A2_S27_assembly & AKR012_contigs) activated sludge metagenome. 1158 S. Yadav, A. Kapley / Science of the Total Environment 692 (2019) 1155–1164

S.Yadry.A Kanley Science of the Total 692 (2019)1155-1164 159 %confidence intervals Protein Metabolism 0 1e-15 Metabolism of Ar tic Co m nds 1e1 Membrane Transpon目 11 1e15 Carbohydrates <1e-15 RNA Metabolism目 <1e-15 Amino Acids and Derivatives <1e-15 Cell Division and Cell Cycle stress Response 1e-15 Cofactors.Vitamins.Prosthetic Groups.Piaments 1e.15 Motility and Chemotaxis日 <1e-15 Nucleosides and Nucleotides -OH <1e-15 Secondary Metabolism <1e-15 Fatty Acids.Lipids.and Isoprenoids O metabolism日 Clustering-based subsystems <1e-15 0 <1e15 Virulence.Disease and Defense <1e-15 Nitrogen Metabolism目 OH <1e15 Cell Wall and Capsule日 0 <1e-15 Regulation and Cell signaling <1e-1 llaneous Proportion (% en pre e ARGs,and MGES 33.Role of mobie genetic elements in the dissemination of ARGs racyie resistance genes were not detected in theA dicated thepr ronments (Chen et al.2016:Guo et al.2017:Wu et al.2018:Zeng compounds.pesticides,and heavy metals may increase the rate of
b). Specifically, ARGs conferring resistance to Aminoglycoside, Betalactam group, erythromycin, methicillin, fluoroquinolones, and lysozyme inhibitors were abundant in both the metagenome. ResFinder 3.1 annotated assembled contigs of both metagenomes using BLAST for acquired resistance genes. In total, 13 and 12 contigs were annotated for acquired resistant genes in A2_S27_assembly and AKR012_contigs respectively. The details are given in Tables 3a and 3b. Acquired ARGs encoding for beta-lactamase, aminoglycoside, rifampicin, trimethoprim, etc. was found in both metagenomes. Genes encoding for resistance like glycopeptide, fosfomycin, macrolide, nitroimidazole, oxazolidinone were not found in both metagenomes. Genes encoding for acquired tetracycline resistance genes were not detected in the A2_S27_assembly metagenome. Similarly, genes encoding for acquired colistin resistance was not found in AKR012_contigs metagenome. Several multidrug ef- flux pumps conferring multidrug resistance including CmeABC_operon which encodes for the multidrug efflux pump in Campylobacter_jejuni were found in both metagenomes. ARGs conferring Methicillin resistance in Staphylococci was also detected. Further genes conferring resistance to toxic metals such as Arsenic, Cadmium, Copper, and Zinc and Mercury were abundant in both sludge metagenome. Metagenomic analyses have shown that antibiotic resistance genes are ubiquitous in every ecosystem including pristine environments (Chen et al., 2016; Guo et al., 2017; Wu et al., 2018; Zeng et al., 2019). Aquatic habitat serves as hotspot exchange of resistant genes. The study confirms that wastewater and WWTPs are among the most significant anthropogenic sources for ARBs, ARGs, and MGEs in the environment. Due to their higher abundance, the selection pressure in such environments is often high which drives the development of new AMR strains (Gullberg et al., 2014; Li et al., 2015; Luo et al., 2017; Pal et al., 2015). 3.3. Role of mobile genetic elements in the dissemination of ARGs Subsystem based analysis of both sludge metagenome data using both MGRAST and STAMP indicated the presence of MGEs, which can be broadly categorized into five types based on their function (Fig. 4). Among the MGEs, sequences encoding for phages and prophages were most abundant, followed by gene transfer agents in both metagenomes. Their abundance may lead to the rapid and broader dissemination of ARGs in the WWTPs and the environment. It can be inferred from the metagenome data that WWTPs and sludge sample act as a hotspot for the selection of antibiotic resistance via horizontal gene transfer (Karkman et al., 2017). MGEs are among important factor which contributes to the dissemination of ARGs. The higher concentration of pharmaceutically active compounds, pesticides, and heavy metals may increase the rate of Fig. 2. Comparative analysis of catabolic diversity present in both activated sludge metagenome using STAMP. S. Yadav, A. Kapley / Science of the Total Environment 692 (2019) 1155–1164 1159

.Yadav.Kapley/Scence ofthe Total Envirorment 6()1155-1164 wwne oua ■ e-er0gv E exc-exD-Op_Matdrug__ pe using KroNa 2016).The evolution and transmission of resistance depend upon the eta2015).Studies have also shown that relative fitness of resistant
lateral gene exchange between pathogenic and nonpathogenic bacteria by HGT in wastewater (Stevenson et al., 2017; von Wintersdorff et al., 2016). The evolution and transmission of resistance depend upon the multiple factors such as rate of mutation, resistance level conferred to the progeny against drug and the strength of selection pressure (Blair et al., 2015). Studies have also shown that relative fitness of resistant Fig. 3. Normalized abundance of the antibiotic resistant genes both activated sludge metagenome using KRONA. 1160 S. Yadav, A. Kapley / Science of the Total Environment 692 (2019) 1155–1164
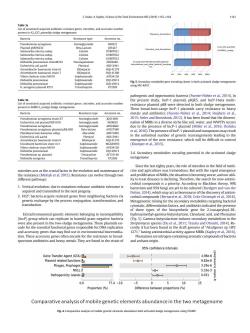
S.Yadry.A Kapley Science of the Total Enyt t62201911155-116 robes.and accession numbe Acces rainM743 strain Mo10 7258 F电5.C-RAST metabolite gene encoding cluster in both activated sludee metagenome pathogenic and bacteria (.1). asmid pB8 we ed in both sludge met m Resistance type Accession no. 7etlandantibiodl Fuet ation of MRB to the presence of In mid n the unlimit number of genetic ding tothe red bi e strain MO10 3.4.Secondary metabolites encoding potential in the activated sludge eighty years,the role prolife tion of A be ent pathways: .and our abi 1.Vertical evolution:due tom nds is a priority.According to Blackbox theory.99 e antibiotic tolerance is utten.2014). ofantim ng for thes ( ompatibility g hyl-gamma ulanic acid,an h 0 o-slu e metag D et al 20 ntly it has pec Tezuka and hnishi.01 ry genes that may find use in environmental bic Te py SoOm ermreo Phenazinesare nitrl activity against MRBs (Kapleyt 95%confidence intervals 98e-8 0 983e8 Phages.Prophage 3,29e-6 0013 0.0 774-10 5 10 15 Proportion (% Difference between proportions (% Comparative analysis of mobile genetic elements abundance in the two metagenome Fig 4.Comparative analysis of mobile genetic elements abundance both activated sludge metagenome using STAMP
microbes acts as the crucial factor in the evolution and maintenance of the resistance (Melnyk et al. 2015). Resistance can evolve through two different pathways: 1. Vertical evolution: due to mutations enhance antibiotic tolerance is acquired and transmitted to the next progeny. 2. HGT: bacteria acquire resistant genes from neighboring bacteria via genetic exchange by the process conjugation, transformation, and transduction. Extrachromosomal genetic elements belonging to incompatibility (IncP) group which can replicate in harmful gram-negative bacteria were also present in the two-sludge metagenome. These plasmids encode for the essential functional genes responsible for DNA replication and accessory genes that may find use in environmental bioremediation. These accessory genes often encode for the resistance to broadspectrum antibiotics and heavy metals. They are found in the strain of pathogenic and opportunistic bacteria (Fuente-Núñez et al., 2014). In the present study, IncP-1 plasmid, pKJK5, and IncP-1beta multiresistance plasmid pB8 were detected in both sludge metagenome. These broad-host-range IncP-1 plasmids carry resistance to heavy metals and antibiotics (Fuente-Núñez et al., 2014; Huijbers et al., 2015; Seiler and Berendonk, 2012). It has been found that the dissemination of MRBs in a diverse niche like soil, water, and WWTPs occurs due to the presence of IncP-1 plasmid (Miller et al., 2016; Osińska et al., 2016). The presence of IncP-1 plasmid and transposons may result in the unlimited number of genetic rearrangements leading to the emergence of the new resistance, which will be difficult to control (Klumper et al., 2015). 3.4. Secondary metabolites encoding potential in the activated sludge metagenome Since the last eighty years, the role of microbes in the field of medicine and agriculture was tremendous. But with the rapid emergence and proliferation of AMRs, the situation is becoming worse, and our ability to treat diseases is declining. Therefore, the search for new antimicrobial compounds is a priority. According to Blackbox theory, 99% bacterium and 95% fungi are yet to be cultured (Bardgett and van der Putten, 2014), which may act as bioresource of the discovery of antimicrobial compounds (Bernard et al., 2018; Crits-Christoph et al., 2018). Metagenomic mining for the secondary metabolites targeting bacterial cytostatic, differentiation factors, and antibiotics indicated the presence of three types of the biosynthetic gene for 2-isocapryloyl-3Rhydroxymethyl-gamma-butyrolactone, Clavulanic acid, and Phenazine (Fig. 5). Gamma-butyrolactone induces secondary metabolism in the Streptomyces species (Du et al., 2011; Tezuka and Ohnishi, 2014). Recently it has been found in the draft genome of “Alcaligenes sp. HPC 1271,” having antimicrobial activity against MRBs (Kapley et al., 2016). Phenazines are nitrogen-containing aromatic compounds of bacteria and archaea origin. Table 3a List of annotated acquired antibiotic resistant genes, microbes, and accession number present in A2_S27_assembly sludge metagenome. Bacteria Resistance type Accession no. Pseudomonas aeruginosa Aminoglycoside X60321 Plasmid pMON234 Beta-Lactam J03427 Salmonella enterica subsp. Colistin KY807921 Salmonella enterica subsp. Colistin KY807922 Salmonella enterica subsp. Colistin KY807923 Klebsiella pneumoniae strainM743 Fluoroquinolone EF636461 Escherichia coli aacA4 Phenicol AB212941 Acinetobacter baumannii strain 6 Rifampicin HQ141279 Acinetobacter baumannii strain 7 Rifampicin HQ141280 Vibrio cholerae strain MO10 Sulphonamide AY034138 Klebsiella pneumoniae Sulphonamide DQ143913 Klebsiella pneumoniae Sulphonamide DQ143914 K. aerogenes plasmid R751 Trimethoprim X72585 Table 3b List of annotated acquired antibiotic resistant genes, microbes, and accession number present in AKR012_contigs sludge metagenome. Bacteria Resistance type Accession no. Pseudomonas aeruginosa strain K7 Aminoglycoside JQ414041 Escherichia coli plasmid RSF1010 Aminoglycoside M28829 Pseudomonas aeruginosa Aminoglycoside AF024602 Pseudomonas aeruginosa plasmid pMLH50 Beta-lactam AY027589 Photobacterium damselae subsp. Macrolide AB571865 Escherichia coli aacA4 Phenicol AB212941 Acinetobacter baumannii strain 6 Rifampicin HQ141279 Uncultured bacterium clone riv1 Sulphonamide MG649393 Vibrio cholerae strain MO10 Sulphonamide AY034138 Klebsiella pneumoniae Sulphonamide DQ143913 Pseudomonas sp. plasmid Tetracycline AF133140 Klebsiella aerogenes Trimethoprim X72585 Fig. 5. Secondary metabolite gene encoding cluster in both activated sludge metagenome using MG-RAST. Fig. 4. Comparative analysis of mobile genetic elements abundance both activated sludge metagenome using STAMP. S. Yadav, A. Kapley / Science of the Total Environment 692 (2019) 1155–1164 1161

a) om both gram e and po e bacteria and Methan s of the dition to this,w etabolit uced by genome anic acid an cteria such as "ESKAP samples, for nove edge about activa resistom cting bl with effluent treatment plants situa 35.Metagenomic analsis using BusyBee Acknowledgment ledee the Director-CSIR-NEERI represent thedistinct Metagenomican india Award No.PDF/2016/0013 stions for imp er Th cked to eCi-NEERUR MacB were also present G-RAST.AS ar SEP/EBGD-DRC/1. Appendix A.Supplementary data oulation and spread of AMR Similarly. analysis of the n38).efflu References Almakki,A.Jumas-Bilak.E.M 4.Conclusion tem func The assembled contigs were analyzed using multiple bioinformatic
They may serve both as an antimicrobial compound having broadspectrum activity and as virulence factors. They have been isolated from both gram-negative and positive bacteria and Methanosarcina species (methanogens) (Blankenfeldt and Parsons, 2014). They also act as cell signaling molecules that regulate the pattern of gene expression and ensure bacterial survival. They exert diverse effects on the eukaryotic host cell leading to the alteration of cellular response. Phenazines are also produced by Pseudomonas aeruginosa, which is an opportunistic pathogen (Pierson and Pierson, 2010). Clavulanic acid was first isolated from Streptomyces clavuligerus. It is composed of “bicyclic β lactam rings” fused to an oxazolidine ring (Paradkar, 2013). It is the potent inhibitor of β lactamases produced from diverse bacteria such as “ESKAPE pathogens” and Shigella. It has lower antibacterial property. In combination with ticarcillin, it is used for the treatment of severe infections affecting blood and internal organs (Demain and Sanchez, 2009). 3.5. Metagenomic analysis using BusyBee BusyBee provides automated clustering/binning reports as a 2D scatter plot (Fig. 6a and b). Each color dot represents an individual sequence. Convex hulls represent the distinct clusters. Metagenomic analysis of the two-metagenome using BusyBee indicated the presence of antibiotic-resistant genes which were not detected previously by MGRAST. Analysis of the metagenome A2_S27_assembly yielded 2 D scatter plot with nine convex hulls indicating the microbial taxonomic abundance. Apart from that sequences encoding for the resistant genes like Vancomycin (van T, van R), Erythromycin (Erm38) were found. Genes encoding for efflux pump like MacB were also present. These resistant genes were not detected in the metagenome using MG-RAST. As we know that vancomycin comes under the category of last resort of antibiotics and its presence in ETP may pose a potential risk to the human population and spread of AMR. Similarly, analysis of the AKR012_contigs metagenome resulted in 2 D scatter plot with 11 convex hulls indicating the microbial taxonomic abundance. Sequences encoding for the resistant genes like Erythromycin (Erm38), efflux pump like MacB, MexC were found. Genes encoding for tetracycline resistance (TetM-TetW) were also detected which were not detected by using MG-RAST. 4. Conclusion Aquatic treatment plants such as ETPs and WWTPs serves as a hot spot for the HGT and dissemination of AMR. Therefore, in the present study, we have analyzed the resistome associated with effluent treatment plants situated in Gujrat, India, using a metagenomic approach. The assembled contigs were analyzed using multiple bioinformatic tools such as MG-RAST, BusyBee, and ResFinder3.1. All the tools indicated the prevalence of diverse resistant genes like tetracycline, sulphonamide, trimethoprim, aminoglycoside, etc. and mobile genetic elements. The resistance profile associated with both effluent treatment plants was found to be similar despite being two different types of the effluent treatment plant. In addition to this, we also analyzed the presence of secondary metabolite genes encoding potential for both metagenomes. The metagenomic analysis confirmed that both metagenomes could encode for biosynthetic gene clusters such as Clavulanic acid and Phenazine biosynthesis. Our ultimate objective will be to construct a functional metagenomic library from pharmaceutical wastewater, pristine environment samples, and to screen for novel secondary metabolites with antimicrobial potential. Overall, the present study expands our current knowledge about activated sludge resistome associated with effluent treatment plants situated in Gujrat, India. Acknowledgment The authors greatly acknowledge the Director-CSIR-NEERI for providing the necessary facility. Shailendra Yadav was supported by National Postdoctoral fellowship funded by Science & Engineering Research Board (SERB), Govt. of India [Award No. PDF/2016/001389]. The authors are incredibly thankful to the reviewers for their valuable suggestions for improving the quality of the paper. The manuscript was checked for the plagiarism using iThenticate software at the NEERI Knowledge resource center: KRC no.: CSIR-NEERI/KRC/2018/ SEP/EBGD-DRC/1. Appendix A. Supplementary data Supplementary data to this article can be found online at https://doi. org/10.1016/j.scitotenv.2019.07.267. References Almakki, A., Jumas-Bilak, E., Marchandin, H., Licznar-Fajardo, P., 2019. Antibiotic resistance in urban runoff. Sci. Total Environ. 667, 64–76. Andersson, D.I., Hughes, D., 2014. Microbiological effects of sublethal levels of antibiotics. Nat Rev Micro 12, 465–478. Band, V.I., Crispell, E.K., Napier, B.A., Herrera, C.M., Tharp, G.K., Vavikolanu, K., et al., 2016. Antibiotic failure mediated by a resistant subpopulation in Enterobacter cloacae. 1, 16053. Bardgett, R.D., van der Putten, W.H., 2014. Belowground biodiversity and ecosystem functioning. Nature 515, 505–511. Berendonk, T.U., Manaia, C.M., Merlin, C., Fatta-Kassinos, D., Cytryn, E., Walsh, F., et al., 2015. Tackling antibiotic resistance: the environmental framework. Nat Rev Micro 13, 310–317. Fig. 6. a) Taxonomic (upto genus level) and functional diversity (ARGs) present in the A2_S27_assembly using BusyBee server. Coloured dots indicates abundance of microbes and the black dots the presence of ARGs. b) Taxonomic (upto genus level) and functional diversity (ARGs) present in the AKR012_contigs using BusyBee server. Coloured dots indicate abundance of microbes and the black dots the presence of ARGs. 1162 S. Yadav, A. Kapley / Science of the Total Environment 692 (2019) 1155–1164
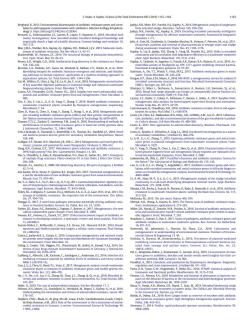
S.Yadry.A Kapley Science of the Totat t62(20191155-1164 1n6回 Jadeja N.B.M alar mech. o20159ni ale.H.Sa LA. V-ctaL2 (MEG)S che AddsW-W iotic resistance in India:drivers and ono L2016 0y50.6670-667 overy.Biochem.Pharmacol.134 organisms as a souro 及C201Me iA-D 13 .2081 2 Pilla.G. he fu very:80 vea x-M.Sha CM.R 2013.The last 0te499394 ForbesJD.KmoxN.C.Ro Pagot etagenomics:the next vak.IT. 10 e13.40 2016 05 13. acid pro by Streptomyces clavuligerus:biogenesi Water Res.123 468-4
Berglund, B., 2015. Environmental dissemination of antibiotic resistance genes and correlation to anthropogenic contamination with antibiotics. Infection Ecology & Epidemiology 5. https://doi.org/10.3402/iee.v5.28564. Bernard, G., Pathmanathan, J.S., Lannes, R., Lopez, P., Bapteste, E., 2018. Microbial dark matter investigations: how microbial studies transform biological knowledge and empirically sketch a logic of scientific discovery. Genome biology and evolution 10, 707–715. Blair, J.M.A., Webber, M.A., Baylay, A.J., Ogbolu, D.O., Piddock, L.J.V., 2015. Molecular mechanisms of antibiotic resistance. Nat Rev Micro 13, 42–51. Blankenfeldt, W., Parsons, J.F., 2014. The structural biology of phenazine biosynthesis. Curr. Opin. Struct. Biol. 29, 26–33. Brown, E.D., Wright, G.D., 2016. Antibacterial drug discovery in the resistance era. Nature 529, 336–343. Brunton, L.A., Desbois, A.P., Garza, M., Wieland, B., Mohan, C.V., Häsler, B., et al., 2019. Identifying hotspots for antibiotic resistance emergence and selection, and elucidating pathways to human exposure: application of a systems-thinking approach to aquaculture systems. Sci. Total Environ. 687, 1344–1356. Cai, M., Wilkins, D., Chen, J., Ng, S.K., Lu, H., Jia, Y., et al., 2016. Metagenomic reconstruction of key anaerobic digestion pathways in municipal sludge and industrial wastewater biogas-producing systems. Front. Microbiol. 7, 778. Castro, A.P., Fernandes, G.D.R., Franco, O.L., 2014. Insights into novel antimicrobial compounds and antibiotic resistance genes from soil metagenomes. Front. Microbiol. 5, 489. Che, Y., Xia, Y., Liu, L., Li, A.-D., Yang, Y., Zhang, T., 2019. Mobile antibiotic resistome in wastewater treatment plants revealed by Nanopore metagenomic sequencing. Microbiome 7, 44. Chen, B., Yuan, K., Chen, X., Yang, Y., Zhang, T., Wang, Y., et al., 2016. Metagenomic analysis revealing antibiotic resistance genes (ARGs) and their genetic compartments in the Tibetan environment. Environmental Science & Technology 50, 6670–6679. Clausen, P.T.L.C., Zankari, E., Aarestrup, F.M., Lund, O., 2016. Benchmarking of methods for identification of antimicrobial resistance genes in bacterial whole genome data. J. Antimicrob. Chemother. 71, 2484–2488. Crits-Christoph, A., Diamond, S., Butterfield, C.N., Thomas, B.C., Banfield, J.F., 2018. Novel soil bacteria possess diverse genes for secondary metabolite biosynthesis. Nature 558, 440. Culligan, E.P., Sleator, R.D., Marchesi, J.R., Hill, C., 2014. Metagenomics and novel gene discovery: promise and potential for novel therapeutics. Virulence 5, 399–412. Dang, B.N., Graham, D.Y., 2017. Helicobacter pylori infection and antibiotic resistance: a WHO high priority? Nat. Rev. Gastroenterol. Hepatol. 14, 383. Das, S., Choudhry, S., Saha, R., Ramachandran, V.G., Kaur, K., Sarkar, B.L., 2011. Emergence of multiple drug resistance Vibrio cholerae O1 in East Delhi. J Infect Dev Ctries 5, 294–298. Demain, A.L., Sanchez, S., 2009. Microbial drug discovery: 80 years of progress. J Antibiot 62, 5–16. dos Santos, D.F.K., Istvan, P., Quirino, B.F., Kruger, R.H., 2017. Functional metagenomics as a tool for identification of new antibiotic resistance genes from natural environments. Microb. Ecol. 73, 479–491. Du, Y.-L., Shen, X.-L., Yu, P., Bai, L.-Q., Li, Y.-Q., 2011. Gamma-butyrolactone regulatory system of Streptomyces chattanoogensis links nutrient utilization, metabolism, and development. Appl. Environ. Microbiol. 77, 8415–8426. Finley, R.L., Collignon, P., Larsson, D.G.J., McEwen, S.A., Li, X.-Z., Gaze, W.H., et al., 2013. The scourge of antibiotic resistance: the important role of the environment. Clin. Infect. Dis. 57, 704–710. Fluegge, K., 2017. A novel host-pathogen interaction potentially driving antibiotic resistance in livestock feedlots. Environ. Sci. Pollut. Res. Int. 24, 12501. Forbes, J.D., Knox, N.C., Ronholm, J., Pagotto, F., Reimer, A., 2017. Metagenomics: the next culture-independent game changer. Front. Microbiol. 8, 1069. Founou, R.C., Founou, L.L., Essack, S.Y., 2017. Clinical and economic impact of antibiotic resistance in developing countries: a systematic review and meta-analysis. PLoS One 12, e0189621. Fuente-Núñez, C., Reffuveille, F., Haney, E.F., Straus, S.K., Hancock, R.E.W., 2014. Broadspectrum anti-biofilm peptide that targets a cellular stress response. PLoS Pathog. 10, e1004152. Gatica, J., Jurkevitch, E., Cytryn, E., 2019. Comparative metagenomics and network analyses provide novel insights into the scope and distribution of β-lactamase homologs in the environment. Front. Microbiol. 10. Göttig, S., Gruber, T.M., Higgins, P.G., Wachsmuth, M., Seifert, H., Kempf, V.A.J., 2014. Detection of pan drug-resistant Acinetobacter baumannii in Germany. J. Antimicrob. Chemother. 69, 2578–2579. Gullberg, E., Albrecht, L.M., Karlsson, C., Sandegren, L., Andersson, D.I., 2014. Selection of a multidrug resistance plasmid by sublethal levels of antibiotics and heavy metals. MBio 5 (e01918-14). Guo, J., Li, J., Chen, H., Bond, P.L., Yuan, Z., 2017. Metagenomic analysis reveals wastewater treatment plants as hotspots of antibiotic resistance genes and mobile genetic elements. Water Res. 123, 468–478. He, L.-Y., He, L.-K., Liu, Y.-S., Zhang, M., Zhao, J.-L., Zhang, Q.-Q., et al., 2019. Microbial diversity and antibiotic resistome in swine farm environments. Sci. Total Environ. 685, 197–207. Hofer, U., 2019. The cost of antimicrobial resistance. Nat Rev Microbiol 17, 3. Holmes, A.H., Moore, L.S., Sundsfjord, A., Steinbakk, M., Regmi, S., Karkey, A., et al., 2016. Understanding the mechanisms and drivers of antimicrobial resistance. Lancet 387, 176–187. Huijbers, P.M.C., Blaak, H., de Jong, M.C.M., Graat, E.A.M., Vandenbroucke-Grauls, C.M.J.E., de Roda Husman, A.M., 2015. Role of the environment in the transmission of antimicrobial resistance to humans: a review. Environmental Science & Technology 49, 11993–12004. Jadeja, N.B., More, R.P., Purohit, H.J., Kapley, A., 2014. Metagenomic analysis of oxygenases from activated sludge. Bioresour. Technol. 165, 250–256. Jadeja, N.B., Purohit, H.J., Kapley, A., 2019. Decoding microbial community intelligence through metagenomics for efficient wastewater treatment. Functional & Integrative Genomics 1–13. Jelic, A., Gros, M., Ginebreda, A., Cespedes-Sánchez, R., Ventura, F., Petrovic, M., et al., 2011. Occurrence, partition and removal of pharmaceuticals in sewage water and sludge during wastewater treatment. Water Res. 45, 1165–1176. Kapley, A., Liu, R., Jadeja, N.B., Zhang, Y., Yang, M., Purohit, H.J., 2015. Shifts in microbial community and its correlation with degradative efficiency in a wastewater treatment plant. Appl. Biochem. Biotechnol. 176, 2131–2143. Kapley, A., Tanksale, H., Sagarkar, S., Prasad, A.R., Kumar, R.A., Sharma, N., et al., 2016. Antimicrobial activity of Alcaligenes sp. HPC 1271 against multidrug resistant bacteria. Functional & integrative genomics 16, 57–65. Karkman, A., Do, T.T., Walsh, F., Virta, M.P.J., 2017. Antibiotic-resistance genes in waste water. Trends Microbiol. 26, 220–228. Keegan, K.P., Glass, E.M., Meyer, F., 2016. MG-RAST, a metagenomics service for analysis of microbial community structure and function. Microbial Environmental Genomics (MEG). Springer, pp. 207–233. Klumper, U., Riber, L., Dechesne, A., Sannazzarro, A., Hansen, L.H., Sorensen, S.J., et al., 2015. Broad host range plasmids can invade an unexpectedly diverse fraction of a soil bacterial community. ISME J 9, 934–945. Laczny, C.C., Kiefer, C., Galata, V., Fehlmann, T., Backes, C., Keller, A., 2017. BusyBee Web: metagenomic data analysis by bootstrapped supervised binning and annotation. Nucleic Acids Res. 45, W171–W179. Laxminarayan, R., Chaudhury, R.R., 2016. Antibiotic resistance in India: drivers and opportunities for action. PLoS Med. 13, e1001974. Lewis, J.D., Chen, E.Z., Baldassano, R.N., Otley, A.R., Griffiths, A.M., Lee, D., 2015. Inflammation, antibiotics, and diet as environmental stressors of the gut microbiome in pediatric Crohn's disease. Cell Host Microbe 18, 489–500. Lewis, K., 2017. New approaches to antimicrobial discovery. Biochem. Pharmacol. 134, 87–98. Lewis, K., Epstein, S., D'Onofrio, A., Ling, L.L., 2010. Uncultured microorganisms as a source of secondary metabolites. J Antibiot 63, 468–476. Li, A.-D., Li, L.-G., Zhang, T., 2015. Exploring antibiotic resistance genes and metal resistance genes in plasmid metagenomes from wastewater treatment plants. Front. Microbiol. 6, 1025. Liu, Y., Yang, D., Zhang, N., Chen, L., Cui, Z., Shen, Q., et al., 2016. Characterization of uncultured genome fragment from soil metagenomic library exposed rare mismatch of internal tetranucleotide frequency. Front. Microbiol. 7, 2081. Lobanovska, M., Pilla, G., 2017. Penicillin's discovery and antibiotic resistance: lessons for the future? The Yale Journal of Biology and Medicine 90, 135–145. Luo, G., Li, B., Li, L.-G., Zhang, T., Angelidaki, I., 2017. Antibiotic resistance genes and correlations with microbial community and metal resistance genes in full-scale biogas reactors as revealed by metagenomic analysis. Environmental Science & Technology 51, 4069–4080. Lv, X.-M., Shao, M.-F., Li, J., Li, C.-L., 2015. Metagenomic analysis of the sludge microbial community in a lab-scale denitrifying phosphorus removal reactor. Appl. Biochem. Biotechnol. 175, 3258–3270. Manaia, C.M., Rocha, J., Scaccia, N., Marano, R., Radu, E., Biancullo, F., et al., 2018. Antibiotic resistance in wastewater treatment plants: tackling the black box. Environ. Int. 115, 312–324. McKenna, M., 2013. The last resort. Nature 499, 394. Melnyk, A.H., Wong, A., Kassen, R., 2015. The fitness costs of antibiotic resistance mutations. Evolutionary applications 8, 273–283. Miller, J.H., Novak, J.T., Knocke, W.R., Pruden, A., 2016. Survival of antibiotic resistant bacteria and horizontal gene transfer control antibiotic resistance gene content in anaerobic digesters. Front. Microbiol. 7, 263. Nnadozie, C., Kumari, S., Bux, F., 2017. Status of pathogens, antibiotic resistance genes and antibiotic residues in wastewater treatment systems. Rev. Environ. Sci. Biotechnol. 16, 491–515. Nowrotek, M., Jałowiecki, Ł., Harnisz, M., Płaza, G.A., 2019. Culturomics and metagenomics: in understanding of environmental resistome. Frontiers of Environmental Science & Engineering 13, 40. Osińska, A., Harnisz, M., Korzeniewska, E., 2016. Prevalence of plasmid-mediated multidrug resistance determinants in fluoroquinolone-resistant bacteria isolated from sewage and surface water. Environ. Sci. Pollut. Res. Int. 23, 10818–10831. Pal, C., Bengtsson-Palme, J., Kristiansson, E., Larsson, D.G.J., 2015. Co-occurrence of resistance genes to antibiotics, biocides and metals reveals novel insights into their coselection potential. BMC Genomics 16, 964. Paradkar, A., 2013. Clavulanic acid production by Streptomyces clavuligerus: biogenesis, regulation and strain improvement. J Antibiot 66, 411–420. Parks, D.H., Tyson, G.W., Hugenholtz, P., Beiko, R.G., 2014. STAMP: statistical analysis of taxonomic and functional profiles. Bioinformatics 30, 3123–3124. Pierson, L.S., Pierson, E.A., 2010. Metabolism and function of phenazines in bacteria: impacts on the behavior of bacteria in the environment and biotechnological processes. Appl. Microbiol. Biotechnol. 86, 1659–1670. Raina, V., Panda, A.N., Mishra, S.R., Nayak, T., Suar, M., 2019. Microbial biodiversity study of a brackish water ecosystem in eastern India: The Chilika Lake. Microbial Diversity in the Genomic Era. Elsevier, pp. 47–63. Reddy, B., Dubey, S.K., 2019. River Ganges water as reservoir of microbes with antibiotic and metal ion resistance genes: high throughput metagenomic approach. Environ. Pollut. 246, 443–451. Seemann, T., 2014. Prokka: rapid prokaryotic genome annotation. Bioinformatics 30, 2068–2069. S. Yadav, A. Kapley / Science of the Total Environment 692 (2019) 1155–1164 1163

1164 S.Yadav.A Kapley/Science of the Total Emviro met692(2019】1155-1164 TR.Weeks Livermore DM.Toleman.MA.0.Dis ination of NDM-1 soils Shd .NM AA AA K e drug-resistant bacteria that pose the greatest health threats Na- ty s plan biol. ilding the web of .CJ.Penders.Jvan Niekerk.JM.Mills.N.D.Majumder.S.van Alphen et al.2016.Di achko.LTop.EM.Linking the resistome and plas Y-CO al du CHaP .W ty pro- X-x Zh Yadav AA Kapley.A2014.sh in microbial tibiotic n ba Pan.Y.Ya Xiong W ent bio mmun Zeng. Hou,M.Zeng. 2019.Metageno be126. Teitze LG2019.R with nove therapeuti Trends .H.He.H.Chen.S.Hu 2019 Abundance ofantibiot Tezuka,T .Microbial ho nce gene and their tion with al dis rom G an,T ites
Seiler, C., Berendonk, T.U., 2012. Heavy metal driven co-selection of antibiotic resistance in soil and water bodies impacted by agriculture and aquaculture. Front. Microbiol. 3, 399. Sen, U., Mukhopadhyay, S.K., 2019. Microbial community composition of saltern soils from Ramnagar, West Bengal, India. Ecological Genetics and Genomics 12, 100040. Shchegolkova, N.M., Krasnov, G.S., Belova, A.A., Dmitriev, A.A., Kharitonov, S.L., Klimina, K.M., et al., 2016. Microbial community structure of activated sludge in treatment plants with different wastewater compositions. Front. Microbiol. 7, 90. Soucy, S.M., Huang, J., Gogarten, J.P., 2015. Horizontal gene transfer: building the web of life. Nat Rev Genet 16, 472–482. Stalder, T., Press, M.O., Sullivan, S., Liachko, I., Top, E.M., 2019. Linking the resistome and plasmidome to the microbiome. The ISME journal 1. Stevenson, C., Hall, J.P.J., Harrison, E., Wood, A., Brockhurst, M.A., 2017. Gene mobility promotes the spread of resistance in bacterial populations. ISME J 11, 1930–1932. Tao, W., Zhang, X.-X., Zhao, F., Huang, K., Ma, H., Wang, Z., et al., 2016. High levels of antibiotic resistance genes and their correlations with bacterial community and mobile genetic elements in pharmaceutical wastewater treatment bioreactors. PLoS One 11, e0156854. Teitzel, G., 2019. Responding to antimicrobial resistance with novel therapeutics. Trends Microbiol. 27, 285–286. Tezuka, T., Ohnishi, Y., 2014. Microbial hormones as a master switch for secondary metabolism in streptomyces. In: Anazawa, H., Shimizu, S. (Eds.), Microbial Production: From Genome Design to Cell Engineering. Springer, Japan, Tokyo, pp. 179–190. Vaishnav, P., Demain, A.L., 2011. Unexpected applications of secondary metabolites. Biotechnol. Adv. 29, 223–229. Walsh, T.R., Weeks, J., Livermore, D.M., Toleman, M.A., 2011. Dissemination of NDM-1 positive bacteria in the New Delhi environment and its implications for human health: an environmental point prevalence study. Lancet Infect. Dis. 11, 355–362. WHO, 2014. Antimicrobial Resistance: Global Report on Surveillance. World Health Organization, Geneva. Willyard, C., 2017. The drug-resistant bacteria that pose the greatest health threats. Nature News 543, 15. von Wintersdorff, C.J., Penders, J., van Niekerk, J.M., Mills, N.D., Majumder, S., van Alphen, L.B., et al., 2016. Dissemination of antimicrobial resistance in microbial ecosystems through horizontal gene transfer. Front. Microbiol. 7. Wu, Y., Cui, E., Zuo, Y., Cheng, W., Chen, H., 2018. Fate of antibiotic and metal resistance genes during two-phase anaerobic digestion of residue sludge revealed by metagenomic approach. Environ. Sci. Pollut. Res. 25, 13956–13963. Yadav, T.C., Khardenavis, A.A., Kapley, A., 2014. Shifts in microbial community in response to dissolved oxygen levels in activated sludge. Bioresource technology 165, 257–264. Zeng, J., Pan, Y., Yang, J., Hou, M., Zeng, Z., Xiong, W., 2019. Metagenomic insights into the distribution of antibiotic resistome between the gut-associated environments and the pristine environments. Environ. Int. 126, 346–354. Zhang, H., He, H., Chen, S., Huang, T., Lu, K., Zhang, Z., et al., 2019. Abundance of antibiotic resistance genes and their association with bacterial communities in activated sludge of wastewater treatment plants: geographical distribution and network analysis. J. Environ. Sci. 82, 24–38. 1164 S. Yadav, A. Kapley / Science of the Total Environment 692 (2019) 1155–1164
按次数下载不扣除下载券;
注册用户24小时内重复下载只扣除一次;
顺序:VIP每日次数-->可用次数-->下载券;
- 甘肃农业大学:食品科学与工程学院(文献讲义)Use of the Secreted Proteome of Trametes versicolor for Controlling the Cereal Pathogen Fusarium langsethiae.pdf
- 甘肃农业大学:食品科学与工程学院(文献讲义)Honey bee(Apis mellifera)larval pheromones may regulate gene expression related to foraging task specialization.pdf
- 甘肃农业大学:食品科学与工程学院(文献讲义)Conventional and omics approaches shed light on Halitzia cheese, a long-forgotten white-brined cheese from Cyprus.pdf
- 甘肃农业大学:食品科学与工程学院(文献讲义)Comparison of bacterial diversity in traditionally homemade paocai and Chinese spicy cabbage.pdf
- 甘肃农业大学:食品科学与工程学院(文献讲义)Transcriptome Changes during Major Developmental Transitions Accompanied with Little Alteration of DNA Methylome in Two Pleurotus Species.pdf
- 甘肃农业大学:《食品加工高新技术》课程教学资源(课件讲义)高新杀菌技术和膜过滤技术.pdf
- 甘肃农业大学:《食品加工高新技术》课程教学资源(课件讲义)超高压技术.pdf
- 甘肃农业大学:《食品加工高新技术》课程教学资源(课件讲义)超微粉碎技术.pdf
- 甘肃农业大学:《食品加工高新技术》课程教学资源(课件讲义)超临界萃取技术及其应用.pdf
- 甘肃农业大学:《食品加工高新技术》课程教学资源(课件讲义)膜分离技术.pdf
- 甘肃农业大学:《食品加工高新技术》课程教学资源(课件讲义)微胶囊化技术.pdf
- 甘肃农业大学:《食品加工高新技术》课程教学资源(课件讲义)食品杀菌新技术.pdf
- 甘肃农业大学:《食品加工高新技术》课程教学资源(课件讲义)食品冷冻新技术.pdf
- 甘肃农业大学:《食品加工高新技术》课程教学资源(课件讲义)绪论 High and New Food Processing Technology(主讲:韩舜愈).pdf
- 甘肃农业大学:《食品加工高新技术》课程教学资源(学业论文)高新技术在葡萄酒产业中的应用.pdf
- 甘肃农业大学:《食品加工高新技术》课程教学资源(学业论文)高新技术在食品可持续生产中的作用.pdf
- 甘肃农业大学:《食品加工高新技术》课程教学资源(学业论文)微胶囊在食品工业中的应用研究.pdf
- 甘肃农业大学:《食品加工高新技术》课程教学资源(学业论文)食品杀菌新技术.pdf
- 甘肃农业大学:《食品加工高新技术》课程教学资源(学业论文)冷冻粉碎技术在食品中的研究与应用.pdf
- 甘肃农业大学:《食品加工高新技术》课程教学资源(学业论文)微波技术在食品工业中的应用及研究进展.pdf
- 中华人民共和国国家标准:食品安全国家标准(GB 4789.15—2010)食品微生物学检验、霉菌和酵母计数 National food safety standard Food microbiological examination:Enumeration of moulds and yeasts.pdf
- 甘肃农业大学:食品科学与工程学院(文献讲义)食品加工新技术——冷冻浓缩.pdf
- 甘肃农业大学:食品科学与工程学院(文献讲义)超高压技术研究及发展.pdf
- 甘肃农业大学:食品科学与工程学院(文献讲义)微胶囊在食品工业中的应用研究.pdf
- 甘肃农业大学:食品科学与工程学院(文献讲义)超临界CO2萃取技术的研究与应用.pdf
- 甘肃农业大学:食品科学与工程学院(文献讲义)高新技术在葡萄酒产业中的应用.pdf
- 甘肃农业大学:食品科学与工程学院(文献讲义)高新技术在葡萄酒加工中的应用.pdf
- 甘肃农业大学:食品科学与工程学院(文献讲义)新技术.pdf
- 甘肃农业大学:食品科学与工程学院(文献讲义)食品高新技术在葡萄酒中的应用.pdf
- 甘肃农业大学:食品科学与工程学院(文献讲义)Exploring flavour-producing core microbiota in multispecies solidstate fermentation of traditional Chinese vinegar.pdf
- 甘肃农业大学:食品科学与工程学院(文献讲义)Investigation of Glutamate Dependence Mechanism for Poly-γglutamic Acid Production in Bacillus subtilis on the Basis of Transcriptome Analysis.pdf
- 甘肃农业大学:食品科学与工程学院(文献讲义)Casing microbiome dynamics during button mushroom cultivation - implications for dry and wet bubble diseases.pdf
- 甘肃农业大学:食品科学与工程学院(文献讲义)Discrepant gene functional potential and cross-feedings of anammox bacteria Ca. Jettenia caeni and Ca. Brocadia sinica in response to acetate.pdf
- 甘肃农业大学:食品科学与工程学院(文献讲义)Selective enrichment of bacterial pathogens by microplastic biofilm.pdf
- 甘肃农业大学:食品科学与工程学院(文献讲义)Viral metagenomic analysis of the cheese surface - A comparative study of rapid procedures for extracting viral particles.pdf
- 甘肃农业大学:食品科学与工程学院(文献讲义)微生物的研究方法 Study Methods of Microbiology.pdf
- 甘肃农业大学:食品科学与工程学院(文献讲义)The potential of cottonseed hull as biorefinery substrate after biopretreatment by Pleurotus ostreatus and the mechanism analysis based on comparative proteomics.pdf
- 甘肃农业大学:食品科学与工程学院(文献讲义)High-throughput sequencing approach to characterize dynamic changes of the fungal and bacterial communities during the production of sufu, a traditional Chinese fermented soybean food.pdf
- 甘肃农业大学:食品科学与工程学院(文献讲义)亚临界流体萃取技术.pdf
- 甘肃农业大学:食品科学与工程学院(文献讲义)实验九 霉菌的形态观察与鉴定(霉菌的无性孢子和有性孢子的形态观察).pdf